The risk of cardiovascular toxicity caused by cancer radiotherapy—a narrative review
Introduction
Radiotherapy (RT), also known as radiation therapy, is an important component of anticancer treatment currently administered to about half of the patients with cancer worldwide (1). The modality has led to a significant improvement in the chances of surviving a diagnosis of cancer. On the other hand, during the course of therapeutic radiation, noncancerous normal tissues might be affected either by direct radiation exposure or by radiation-induced bystander effect, resulting in radiation-induced toxicity which sometimes become clinically evident years or decades after completion of therapy (2,3).
It has long been known that high doses of radiation like those given during mediastinal RT may damage cardiovascular health (4,5). A potential short- or long-term complication of cancer RT involving the heart and circulation is so-called radiation-induced cardiovascular toxicity. A range of cardiovascular complications have been recognized as cardiovascular toxicity caused by cancer RT, including pericarditis, valvular disease, arrhythmias, ischemic heart disease, coronary artery diseases, cardiomyopathy and heart failure (6-8). In fact, complications can be seen with any dose. The number of cancer survivors who diagnosed with premature heart disease is increasing despite the patients with no preexisting cardiovascular disease or presence of other cardiac risk factors such as hypertension, dyslipidemia, and diabetes mellitus (9).
Nowadays, despite an awareness of the potential cardiotoxicity of irradiation, leading to the application of improved RT techniques and modalities that reduce the volume of normal tissue to be exposed to high doses of radiation compared with conventional therapies, the risk of subsequent heart disease remains an issue among cancer survivors (10-12). Because the biological mechanisms of harm are very complex and only partially understood, radiation oncologists face the challenge of treating patients with the best strategies without adversely impacting cardiovascular health. This review summarizes the current literature regarding the basic pathogenic mechanisms, development and prevention of radiation-induced cardiovascular toxicity. We present the following article in accordance with the Narrative Review reporting checklist (available at https://tro.amegroups.com/article/view/10.21037/tro-21-34/rc).
Methods
We performed a literature search on PubMed to find reported histological observations and studies on acute and late cardiovascular toxicity in cancer patients treated with RT (Table 1). A systematized review of articles on the present topic published between May 1967 and April 2021 was carried out.
Table 1
Items | Specification |
---|---|
Date of Search (specified to date, month and year) | 28 April 2021 |
Databases and other sources searched | PubMed |
Search terms used (including MeSH and free text search terms and filters) | The search strategy used on PubMed included the following terms: ‘radiation’, ‘cancer’, ‘cardiac’, ‘heart’, ‘cardiovascular toxicity’, ‘heart disease’, ‘breast cancer’, ‘lung cancer’, ‘Hodgkin’s lymphoma’, ‘radiotherapy’, ‘modern radiotherapy technique’, ‘cardiac toxicity’, ‘echocardiography’, ‘radiation therapy’, ‘cardiotoxicity’ |
Timeframe | May 1967 to April 2021 |
Inclusion and exclusion criteria (study type, language restrictions etc.) | Inclusion: publish in English or German languages |
Selection process (who conducted the selection, whether it was conducted independently, how consensus was obtained, etc.) | Dai-Wei Liu, Yen-Rong Fu, Shu-Hsin Liu conducted the selection independently and performed data analysis and interpretation. All authors discussed to reach a consensus |
Any additional considerations, if applicable | None |
We included literature data on case studies of cardiovascular toxicity after cancer RT, mainly from survivors of breast cancer and Hodgkin’s lymphoma, risk factors, pathogenesis, development of cardiovascular toxicity after RT, and modern RT techniques utilized to reduce cardiac radiation dose. Included studies were limited to publish in English or German languages.
The search strategy used on PubMed included the following terms: ‘radiation’, ‘cancer’, ‘cardiac’, ‘heart’, ‘cardiovascular toxicity’, ‘heart disease’, ‘breast cancer’, ‘lung cancer’, ‘Hodgkin’s lymphoma’, ‘radiotherapy’, ‘modern radiotherapy technique’, ‘cardiac toxicity’, ‘echocardiography’, ‘radiation therapy’, ‘cardiotoxicity’.
Risk factors
Risk factors of radiation induced cardiovascular toxicity have been investigated. A major risk factor for subsequent development of cardiovascular toxicity is the total dose of mediastinal radiation received (13). The dose of radiation per fraction, the volume of heart irradiated, and the extent to which the coronary arteries are exposed in the radiation field are also critical risk factors for development of cardiovascular toxicity (9,14,15). Generally, radiation doses are fractionated into smaller daily doses of <2 Gy because it has been suggested that fractions of >3 Gy are implicated in a greater risk of developing cardiotoxicity, particularly pericardial effusions (15). In addition, concomitant use of cardiotoxic chemotherapy agents, typically 5-fluorouracil, trastuzumab, and anthracyclines further increase the risk of heart disease (16). Other risk factors include younger age at the time of radiation treatment, preexisting cardiovascular disease, presence of cardiac risk factors (hypertension, dyslipidemia, diabetes, smoking and obesity) and genetic susceptibility (6).
Radiation associated cardiotoxicity appears to be delayed—usually 10 to 40 years following treatment, but acute cardiac inflammation can occur at the time of RT or shortly afterwards, resulting in myocarditis or pericarditis (17). Establishment of models for early risk stratification may help to identify high-risk patients. Modification of RT and administration of preventive treatment will be needed for high-risk patients to reduce the risk of cardiovascular toxicity caused by cancer RT.
Case studies of cardiovascular toxicity after cancer RT
In 1967, the first comprehensive series of heart disease following radiation was reported by Cohn et al. (18). Since then cardiac involvement after mediastinal radiation therapy has been increasingly described. Table 2 listed a summary of the selected histological observations and studies of cancer RT reported in the first century. The literature on the late cardiovascular toxicity of RT comes mainly from survivors of breast cancer and Hodgkin’s lymphoma, diseases in which RT is a common modality of the initial management and in which survival is often prolonged. Similar cardiovascular toxic effects may be present in patients with lung cancer, head and neck cancer and esophageal cancer who receive thoracic RT while data are more limited (24-26).
Table 2
Year | Neoplasm | Treatment | Description | Ref. |
---|---|---|---|---|
1984 | Hodgkin’s disease | 3,000 rad or more to the mediastinum | A significant increase in the incidence of pericarditis with an increased dose of radiation at 2 cm, 5 cm and midplane depths and also with the presence of a large intrathoracic tumor | (19) |
1987 | Hodgkin’s disease Lymphoma Breast carcinoma Cystic hygroma | 42±7 Gy | CAD developed in 15 patients at a mean of 16 years (range, 3–29 years) after chest irradiation. At least 50% diameter narrowing of the left main coronary artery and severe ostial stenosis of the right coronary artery were observed in some cases by coronary angiography. Valvular heart disease, pericardial disease and complete heart block were found in some cases. Severe mediastinal and pericardial fibrosis were presented in 3 patients | (20) |
1989 | Breast carcinoma | Immediate post-operative radiotherapy (radiated group) or delayed radiotherapy on recurrence (watched group) | There was a significantly increased mortality in the radiated group. After 15 years. The relative risk after 15 years for the radiated group relative to the watched group was 1.43 with a 95% confidence interval of 1.13 to 1.81. This increased mortality was correlated to deaths from cardiovascular disease | (21) |
1991 | Hodgkin's disease | 25 MV photons from a linear accelerator | Of the 499 patients, 35 pericarditis (10-year cumulative incidence rate of 9.5%) and 13 myocardial infarctions (10-year cumulative incidence rate of 3.9%). The pericarditis risk was significantly increased with total dose greater than or equal to 41 Gy and with dose per fraction greater than or equal to 3.0 Gy | (22) |
1993 | Hodgkin’s disease Lymphoma Breast Cancer Seminoma | 40–122 Gy | Onset of clinical signs from 3 to 28 years (mean 12), including aortic stenosis, pericardial effusion, constrictive pericarditis, mitral/tricuspid regurgitation, myocardial infraction, mitral regurgitation, or mitral stenosis/regurgitation. The effect of chest irradiation could be a reason leading the young patients who free from risk factors to development of CAD | (23) |
CAD, coronary artery disease.
Studying the risk of the radiation-induced cardiovascular toxicity after RT for lung cancer are more complex than others for several reasons. First, patients with lung cancer are old at diagnosis (average 71-year-old) and most have multiple comorbidities including cardiovascular diseases, chronic obstructive pulmonary diseases, diabetes and other malignancies (27-29). Second, the prognosis of patients with lung cancer is poor. The 5-year survival rate for all patients with lung cancer worldwide is 10% to 20% (30). Third, patients with lung cancer have shown a high prevalence of concomitant cardiac disease (approximately 25% to 30%) (27). Preexisting cardiovascular comorbidities have been involved in increased incidence of cardiovascular events and mortality. Sometimes a proportion of lung cancer patients can be treated curatively due to advances in RT, but the dose to the heart in curative-intent lung RT is usually larger than that for lymphoma or breast cancer; some patients received high-dose conformal RT with concurrent chemotherapy (31). Despite a non-negligible risk of complications- the relatively higher heart dose exposure for lung cancer leading to the earlier onset of cardiovascular toxicity, the benefits of application of radiation outweigh its risks in selected cases. Therefore, much work remains to further refine the strategies of cancer RT, and it is suggested that future research with the evidence around cardiovascular toxicity of lung cancer RT can be separated from previous evidence from other cancer sites (27).
Recent thirty years a number of research groups conducted prospective cohort studies to assess the risk of cardiovascular toxicity caused by cancer RT, with a large study population that make it possible to estimate relative and absolute risk of radiation-related heart disease. Selected key studies are described as followed.
Breast cancer
Early studies with RT in breast cancer patients uncovered the correlation of incidental radiation to the heart with an increase in the frequency of cardiovascular disease. Darby SC and colleagues conducted a population-based case-control study of major coronary events in 2,168 women in Sweden and Denmark who underwent RT for breast cancer between 1958 and 2001. They found that incidental exposure of the heart to radiation treatment for breast cancer elevated the rate of major coronary events (i.e., coronary revascularization, myocardial infarction, or death from ischemic heart disease) by 7.4% for each increase of 1 Gy in the mean radiation dose delivered to the heart (13). Moreover, in the study, women with and women without preexisting cardiac risk factors have a similar proportional increase in the rate of major coronary events per gray (13).
The potential damage to the heart is correlated to the heart-absorbed dose and differs between left- and right-breast radiation therapies. The mean cardiac dose from irradiation of a left-sided breast cancer can be 2 or 3 times that for a right-sided breast cancer (32). As a consequence, long-term mortality from heart disease is increased among women with left-sided breast tumors compared with women with right-sided breast tumors. A prospective cohort study reported by Darby et al. have shown that, in women recorded in the US Surveillance Epidemiology and End Results (SEER) cancer registries as having been diagnosed with breast cancer during 1973–1982 and received radiation, the cardiac mortality ratio, left versus right tumor laterality, was during the periods 10–14 years (1.42) and 15 years or more (1.58) after diagnosis, significantly greater than 1.00 (33).
As described above, accumulated studies and clinical evidence indicated that cardiac mortality and morbidity of breast cancer survivors treated with conventional RT was increased, and thus, for chronic cardiac events in breast cancer patients treated until the 1990s, RT was referred as a risk factor (34). However, improved modern techniques minimize heart doses and reduce radiation exposure of the heart. Merzenich and colleagues investigated cardiac mortality and morbidity of breast cancer survivors treated with contemporary RT in Germany (34). A total of 11,982 breast cancer patients treated between 1998 and 2008 were included in the retrospective cohort study. They found that, after a median follow-up time of 11.1 years, there was no significant correlation between tumor laterality and cardiac mortality in irradiated patients and tumor laterality was not a strong risk factor for cardiac morbidity. However, longer follow-up is needed for assessment of clinically manifest late cardiac effects of cancer RT (34).
Hodgkin’s lymphoma (HL)
HL survivors are at relative high risk of cardiovascular diseases and serve as a model for radiation-induced heart diseases because higher radiation doses to the mediastinum are utilized for HL treatment compared to other cancers (7). A retrospective study of 2,232 patients with HL who received mediastinal irradiation from 1960 through 1991 reported that, in comparison with a matched general population, total doses of >30 Gy led to a 3.5-fold higher risk for cardiac death (35). The study further assessed the risk factors of death from heart disease after Hodgkin’s disease therapy by comparing treated patients with a matched general population. The results indicated that high mediastinal doses, young age at irradiation, lack of protective cardiac blocking, and the increased length of follow-up time were the risk factors of subsequent death from heart disease (35).
In 2015, van Nimwegen FA and colleagues conducted a retrospective cohort study to examine relative and absolute excess risk up to 40 years since HL treatment compared with cardiovascular disease incidence in the general population (36). The study included 2,524 Dutch patients who received treatment for HL, including prescribed mediastinal RT dose and anthracycline dose, at younger than 51 years from 1965 through 1995. They found that after 35 years or more, patients still had a 4- to 6-fold increased standardized incidence ratio of coronary heart disease (CHD) or congestive heart failure (HF) compared with the general population. Moreover, patients who received mediastinal RT had a 40-year cumulative incidence of any cardiovascular disease of 54.6% compared with 24.7% in patients not treated with mediastinal RT or anthracyclines (36).
Pathogenesis
RT is the use of ionizing radiation (IR) to eradicate a tumor. Principally, high-energy radiation is deposited on the tumor site to damage the genome of cancer cell and destroy their ability to divide and grow. When living cells are exposed to IR, a burst of excess reactive oxygen species (ROS) is immediately produced and targets molecules such as DNA, proteins, and lipids. This can induce DNA damage and thereafter cell death (37). Ideally, doses are delivered only to the targeted cancer cells, but the physical nature of radiation beams as well as current techniques make this impossible. Therefore, normal tissues are usually exposed or affected by RT, followed by induction of DNA damage, inflammatory responses and oxidative stress, leading to toxicity. This accounts for acute and long-term adverse effects seen with radiation treatment (4,38,39).
The absorption of IR by normal tissue can disrupt DNA structures and produce DNA breaks that activates DNA repair protein ATM. Meanwhile, a sharp increase in ROS through radiolysis of water results in oxidative stress and initiation of a series of molecular and biological signaling events that may repair the damage or injury as well as culminate in permanent physiological changes or cell death (40). Both of activation of ATM and oxidative stress trigger the nuclear factor-kappa B (NF-κB) signaling pathway and inflammatory responses (41,42). Many genes related to the NF-κB signaling pathway were found to be dysregulated even years after radiation (43). NF-κB activation led to sustained inflammation in irradiated human arteries that can explain cardiovascular disease years after radiation exposure (43).
The persistent increase of inflammation markers ICAM-1 and VCAM-1 was observed when comparing time kinetics of radiation-induced changes of inflammatory markers (PECAM-1, ICAM-1, ICAM-2, VCAM-1) in heart microvascular endothelial cells (ECs) (44). When mice received local thorax irradiation with a single dose of 8 Gy, ICAM-1 and VCAM-1 remained up-regulated 20 weeks after irradiation in heart ECs. The late inflammatory responses in heart ECs implied the predisposition for the development of atherosclerotic plaques in heart ECs at later time points (44).
Numerous processes occur, including activation of DNA repair and signaling mechanism, expression of radiation response genes, increased proliferation, and initiation and perpetuation of inflammation, in response to DNA damage to recover the damaged cell, tissue, or organism after radiation exposure. However, these pathways may also play a role in the development of toxicity. The progeny of the irradiated cells may express a high frequency of gene mutations, chromosomal aberrations and cell death. These effects are collectively known as radiation-induced genomic instability (45). Another effect which is proved to be existed outside of radiation field, in non-irradiated cells, is called radiation-induced bystander effect (3). After radiation exposure, free radicals, immune system molecules, expression changes of some genes involved in inflammation and epigenetic factors are produced in irradiated area and may affect nearby non-irradiated cells. Subsequently, process of gene expression, translation, cell proliferation, apoptosis and cells death in nearby non-irradiated cells may be changed. These changes are demonstrated by results of some in vivo studies (46-48). The induction of bystander effects and instabilities may cause a non-specific inflammatory-type response and injury and be implicated in various pathological consequences of radiation exposures (45).
Development of cardiovascular toxicity after RT
The symptoms of radiation-induced cardiovascular toxicity usually require a long incubation period to manifest (18,20,22,23,42,49-53) (Figure 1). It is believed that endothelial cell senescence results from DNA damage and oxidative stress caused by radiation is an initial key pathophysiologic step for development of radiation-induced cardiovascular toxicity (6). After exposure to IR, the damaged cells with double-stranded DNA breaks (DSBs) may continue to divide a limited number of times before undergoing mitotic or apoptotic cell death. Remarkably, malignant transformation and subsequent malignancies may occur years or decades after RT due to some DSBs-affected tumor suppressor genes and cell cycle signaling pathways (2,54).
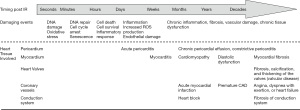
In addition, in response to cellular stress, insulin-like growth factor 1 receptor (IGF-1R) signaling cascade is activated and promotes accelerated senescence in irradiated endothelial cells (55). A decrease in nitric oxide bioavailability, premature senescence and mitochondrial dysfunction in endothelial cells resulted from oxidative stress lead to endothelial dysfunction and inflammatory changes in the radiation field (10).
Senescent endothelial cells with chromosomal aberrations and rearrangements release proteins and proinflammatory cytokines (49,55,56). Elevated levels of IL-1, IL-6, IL-8, and TNF-α as well as ROS (superoxide and peroxynitrite) have all been involved as mediators of the subsequent processes, explaining the latency of acute side effects (2,42,57). Senescent endothelial cells with altered morphology do not proliferate but they stay metabolically active such as oxidative metabolism. This may linked to prolonged oxidative stress (58). Importantly, oxidative changes may continue to arise for days and months after the initial exposure not only in the irradiated cells but also in their progeny (58).
Accumulated evidence suggests that radiation-induced cardiovascular toxicity is a result of various mechanisms interacting with each other through multiple complex signaling pathways (4,38,39). Radiobiology research reveals that normal tissue injury is a dynamic and progressive process (59). As a consequence, chronic inflammation occurs and subsequent fibrosis develops that contribute to structural changes of the heart, such as pericardial inflammation (pericarditis), fibrosis of conduction system, chronic development of fibrosis in the myocardium, endothelial damage in the coronary vessels, and valvular heart diseases (5,51,60,61). A short-term complication of RT, acute pericarditis, is rare but may occur during or days to weeks after irradiation while chronic pericardial effusion or constrictive pericarditis may develop months or years later (62,63). Radiation-related myocardial fibrosis can occur asymptomatically for over 10 years before becoming clinically apparent. The diagnosis of radiation-induced coronary artery disease (CAD) often extends to decades, between radiation exposure and development of obstructive coronary disease (20,23). The majority of patients present with angina, dyspnea with exertion, or heart failure which are traditional symptoms of coronary obstruction. Fibrosis of conduction system is one of the main reasons for developing arrhythmias in later life. Valvular disease is a late complication. Fibrosis, calcification, and thickening of the valves is often asymptomatic but can be diagnosed late, at least 15 years after the original treatment (6). Intimal thickening, lipid deposition, and adventitial fibrosis were observed within the vascular system after irradiation in patients as well as animal studies (64). These changes also follow external irradiation to other parts of the body. All these changes, seen in atherosclerosis and with the normal aging process, accelerate the damage to the vascular endothelium. Atherosclerosis is also worsened by IR (64).
The microvasculature of the myocardium are damaged by presently-used doses of radiation (65). The main cause is inflammatory changes in the microvasculature in response to radiation damage to the myocardium, leading to microthrombi and occlusion of vessels, reduced vascular density, perfusion defects and focal ischemia (53). Subsequently, progressive myocardial cell death and fibrosis can occur. Moreover, capillary network damage is appeared to be irreversible, though endothelial cells can regenerate (38). Biologically, cardiac capillary endothelial cells exposure to radiation can result in their proliferation, swelling and degeneration, injury, and dramatically reduce the number of capillaries (66). This may lead to reduction of the blood supply of myocardium. In addition, the myocardium is particularly vulnerable to oxidative activity of free radicals produced by IR due to the low antioxidant capacity (5).
The primary and fundamental cause of myocardial injury is thought to be radiation-induced endothelial cell injury (38). The thresholds for injury are not fully elucidated. The extent of structural damage to a tissue depends on cell radiosensitivity. Mammalian cardiomyocytes are thought to be relatively resistant to radiation because most of them lose the capacity to undergo cell division shortly after birth (67). However, because of, at least partly, the quite limited proliferative capacity, once tissues in cardiomyocyte are damaged, loss is hardly reconstituted and is irreversible. This frequently results in diminished cardiac function and severe heart failure (67). In addition, cardiomyocyte membrane is rich in phospholipids which are particularly sensitive to oxidative stress. Lipid peroxidation in cardiomyocyte membrane can also lead to functional and structural injury (5).
As mentioned above, radiation-induced cardiovascular toxicity can lead to long-term adverse outcomes, with the median time to diagnosis of radiation-related heart disease being approximately 19 years (65). Besides, damage mechanisms in heart irradiation are affected in a dose-dependent manner. Post-radiation-induced mortality is significantly increase with higher radiation doses, particularly dose >40 Gy. In addition, damaging effects can also be observed even after applying doses as low as 2 Gy (65).
Detection of cardiac toxicity
Echocardiography is a type of ultrasound scan wildly used in the evaluation of cardiac toxicity during and after RT (68-70). It is a noninvasive method of examining the structure and function of the heart and nearby blood vessels (71). Left ventricular ejection fraction (LV-EF) obtained using 2D echocardiography or 3D echocardiography and global longitudinal strain (GLS) measurement based on 2D speckle-tracking echocardiography have been used to evaluate radiation-induced cardiac toxicity (69,71-73). Reduction in LVEF and/or GLS reduction >10% have been considered clinically relevant and referred to subclinical LV dysfunction (69,73,74). Doppler echocardiography is also widely used to monitor cardiotoxicity and identify the main forms of cardiac complications, such as left ventricular (systolic and diastolic) dysfunction, pericarditis and pericardial effusion, valve heart disease, carotid artery lesions, of cancer therapy (70). Use of myocardial strain imaging by echocardiography is critical for the early detection of cardiovascular toxicity in patients during and after cancer RT. Another common noninvasive method chosen for evaluation and management in patients with known or suspected cardiac complications, especially coronary artery disease, is nuclear myocardial perfusion imaging (MPI) with single-photon emission tomography (SPECT) or positron emission tomography (PET) (61,75,76). A controlled clinical head-to-head comparative study showed that PET exhibited higher accuracy and higher sensitivity for diagnosis of myocardial ischemia compared with that of SPECT, whereas SPECT revealed better specificity than PET (75).
Protection against radiation-induced cardiovascular toxicity
Patients with thoracic cancers receiving radiation treatment often involve some incidental exposure of the heart to IR, leading to a late cardiovascular toxic effect which negatively affects quality of life. Unfortunately, prospective studies in the modern era hardly determine their long-term impact on cardiovascular health and therefore no precise program is available for effective eradication of the onset and subsequent development of radiation-induced cardiovascular toxicity. Currently, reducing heart exposed range and minimizing the radiation dose given have become a recognized primary strategy. Improvements in RT planning have reduced the volume of the heart and major coronary vessels to be exposed to high doses. Advanced RT delivery modalities include prior computed tomography (CT)-guided field planning, three-dimensional conformal radiotherapy (3D-CRT) intensity-modulated radiation therapy (IMRT), volumetric modulated arc therapy (VMAT), proton therapy, and respiratory-gated radiotherapy in deep-inspiration breath-hold (DIBH) (77-79). In order to minimize heart doses over a course of RT, DIBH were developed for a more favorable position of the heart during inspiration (80). The patient is either instructed to hold his or her breath at certain points in the breathing cycle, or use devices named as active breathing control (ABC) to hold their breath to maintain the volume. DIBH serving a tool for cardiac sparing during irradiation can produce a significantly lower mean heart dose (80-82). A number of studies have investigated the effects of modern RT techniques (Table 3). The studies suggest that tangential intensity-modulated radiotherapy (t-IMRT) and proton therapy (PT)-DIBH plans showed a significant reduction in mean cardiac dose than VMAT-DIBH whereas as VMAT-DIBH found to benefit a select group of patients (82-87).
Table 3
Cancer type | Number of patients | Treatment type | Outcome | Ref. |
---|---|---|---|---|
Left-sided breast cancer | 15 | t-IMRT- deep- DIBH; VMAT-DIBH; t-IMRT- FB; VMAT-FB | MHD was 5±2.4 Gy, 5.7±1.4 Gy, 9.7±3.3 Gy and 8.1±2.0 Gy for t-IMRT-DIBH, VMAT-DIBH, IMRT-FB and VMAT-FB respectively. On average, there was no significant difference in MHD between VMAT-DIBH and t-IMRT-DIBH. However, VMAT-DIBH was found to benefit a select group of patients | (83) |
Left-sided breast cancer | 3D-CRT or VMAT, in FB or DIBH | 3D-CRT plans in DIBH pose the lowest risk for major coronary events | (84) | |
Left-sided breast cancer | 25 | IMRT- DIBH; VMAT-DIBH; IMRT- FB; VMAT-FB | Both IMRT and VMAT techniques in the DIBH position achieved a considerable dose sparing to the heart and LADCA. Additionally, in comparison with VMAT, both IMRT plans produced a significantly lower mean heart dose | (85) |
Left-sided breast cancer | 20 | DIBH-t-IMRT and DIBH-t-VMAT plans | T-IMRT showed a significant reduction in mean cardiac dose of 26%, in mean dose to LADCA of 20%, in normal tissue integral dose of 19% and the V5% of total body of 24% compared to t-VMAT. EAR for the induction of secondary tumors was significantly lower in the contralateral lung and breast following t-IMRT than t-VMAT. However, t-VMAT achieved better homogeneity and conformity | (82) |
Early-stage, mediastinal HL | 27 | Chemotherapy and INRT delivered as 3D-CRT, VMAT, or PT, compared with the extensive MF | 3D-CRT, VMAT or PT significantly lower the dose to the heart, lungs and breasts and provide lower risk estimates compared with MF, but with substantial patient variability. The risk of cardiovascular disease is not significantly different for 3D-CRT versus VMAT | (86) |
Lower mediastinal lymphoma | 21 | FB-IMRT, DIBH-IMRT, FB-PT, and DIBH-PT plans | Both PT plans produced a significantly lower mean dose to the lung, heart, left ventricle, esophagus, and nontarget body than DIBH-IMRT. DIBH-PT reduced the median MHD by 4.2 Gy (P<0.0001); left ventricle dose by 5.1 Gy (P<0.0001); and lung V5 by 26% (P<0.0001) versus DIBH-IMRT. The 2 PT plans were comparable, with DIBH-PT reducing mean lung dose (7.0 vs. 7.7 Gy; P=0.063) and with no difference in MHD (10.3 vs. 9.5 Gy; P=0.992) | (87) |
INRT, involved node radiotherapy; 3D-CRT, 3D conformal radiotherapy; VMAT, volumetric modulated arc therapy; PT, proton therapy; MF, Mantle Field; t-IMRT, tangential intensity-modulated radiotherapy; DIBH, deep-inspiration breath-hold; FB, free-breathing; MHD, mean heart dose; EAR, excess absolute risk; LADCA, left anterior descending coronary artery.
Attention to reducing the risk of cardiovascular disease should be a priority for the long-term care of patients, especially children, adolescents, and young adults, following the diagnosis and treatment of thoracic cancer.
Conclusions
RT has been used to treat carcinomas for over a century. Cancer survivors who receive thoracic RT in the initial treatment carry a higher risk of developing cardiovascular toxicity (6). Reduction of total heart dose (mean/median) is critical as it is importantly correlated with late cardiac toxicity. Mean heart dose (MHD) over 10 Gy can significantly increase the risk of cardiovascular toxicity after radiation therapy (88). Thus, RT delivery modalities have advanced and additional heart shielding techniques are used. Several different clinical conditions such as pericarditis, cardiomyopathy, coronary syndrome, conduction abnormalities, heart failure, and valvular disease can result from radiation-induced cardiovascular toxicity. None of these conditions is restricted to radiation exposure, but it seems that the pathogenesis of these symptoms is accelerated significantly by irradiation. The exact detail and pathophysiology of radiation-induced cardiovascular toxicity are largely unknown. However, modern irradiation techniques in combination with cardio‐oncology have to develop to reduce the risk of cardiac toxicities and optimize the care of patients with cancer.
Acknowledgments
Funding: This study was supported by the Hualien Tzu Chi Hospital, Buddhist Tzu Chi Medical Foundation (TCRD109-31-HHC; TCRD109-23-HHC).
Footnote
Provenance and Peer Review: This article was commissioned by the Guest Editors (Pei-Wei Shueng, Yen-Wen Wu and Long-Sheng Lu) for the series “Cardio-Oncology” published in Therapeutic Radiology and Oncology. The article has undergone external peer review.
Reporting Checklist: The authors have completed the Narrative Review reporting checklist. Available at https://tro.amegroups.com/article/view/10.21037/tro-21-34/rc
Conflicts of Interest: All authors have completed the ICMJE uniform disclosure form (available at https://tro.amegroups.com/article/view/10.21037/tro-21-34/coif). The series “Cardio-Oncology” was commissioned by the editorial office without any funding or sponsorship. The authors have no other conflicts of interest to declare.
Ethical Statement: The authors are accountable for all aspects of the work in ensuring that questions related to the accuracy or integrity of any part of the work are appropriately investigated and resolved.
Open Access Statement: This is an Open Access article distributed in accordance with the Creative Commons Attribution-NonCommercial-NoDerivs 4.0 International License (CC BY-NC-ND 4.0), which permits the non-commercial replication and distribution of the article with the strict proviso that no changes or edits are made and the original work is properly cited (including links to both the formal publication through the relevant DOI and the license). See: https://creativecommons.org/licenses/by-nc-nd/4.0/.
References
- Delaney G, Jacob S, Featherstone C, et al. The role of radiotherapy in cancer treatment: estimating optimal utilization from a review of evidence-based clinical guidelines. Cancer 2005;104:1129-37. [Crossref] [PubMed]
- Majeed H, Gupta V. Adverse Effects Of Radiation Therapy. StatPearls. Treasure Island (FL): StatPearls Publishing, 2021.
- Najafi M, Fardid R, Hadadi G, et al. The mechanisms of radiation-induced bystander effect. J Biomed Phys Eng 2014;4:163-72. [PubMed]
- Sylvester CB, Abe JI, Patel ZS, et al. Radiation-Induced Cardiovascular Disease: Mecha-nisms and Importance of Linear Energy Transfer. Front Cardiovasc Med 2018;5:5. [Crossref] [PubMed]
- Tapio S. Pathology and biology of radiation-induced cardiac disease. J Radiat Res 2016;57:439-48. [Crossref] [PubMed]
- Koutroumpakis E, Palaskas NL, Lin SH, et al. Modern Radiotherapy and Risk of Cardio-toxicity. Chemotherapy 2020;65:65-76. [Crossref] [PubMed]
- Marmagkiolis K, Finch W, Tsitlakidou D, et al. Radiation Toxicity to the Cardiovascular System. Curr Oncol Rep 2016;18:15. [Crossref] [PubMed]
- Yusuf SW, Venkatesulu BP, Mahadevan LS, et al. Radiation-Induced Cardiovascular Disease: A Clinical Perspective. Front Cardiovasc Med 2017;4:66. [Crossref] [PubMed]
- Belzile-Dugas E, Eisenberg MJ. Radiation-Induced Cardiovascular Disease: Review of an Underrecognized Pathology. J Am Heart Assoc 2021;10:e021686. [Crossref] [PubMed]
- Baselet B, Rombouts C, Benotmane AM, et al. Cardiovascular diseases related to ionizing radiation: The risk of low-dose exposure Int J Mol Med 2016;38:1623-41. (Review). [Crossref] [PubMed]
- Lim YJ, Koh J. Heart-related mortality after postoperative breast irradiation in patients with ductal carcinoma in situ in the contemporary radiotherapy era. Sci Rep 2021;11:2790. [Crossref] [PubMed]
- Lipshultz SE, Adams MJ, Colan SD, et al. Long-term cardiovascular toxicity in children, adolescents, and young adults who receive cancer therapy: pathophysiology, course, monitoring, management, prevention, and research directions: a scientific statement from the American Heart Association. Circulation 2013;128:1927-95. [Crossref] [PubMed]
- Darby SC, Ewertz M, McGale P, et al. Risk of ischemic heart disease in women after ra-diotherapy for breast cancer. N Engl J Med 2013;368:987-98. [Crossref] [PubMed]
- van Nimwegen FA, Schaapveld M, Cutter DJ, et al. Radiation Dose-Response Relationship for Risk of Coronary Heart Disease in Survivors of Hodgkin Lymphoma. J Clin Oncol 2016;34:235-43. [Crossref] [PubMed]
- Martel MK, Sahijdak WM, Ten Haken RK, et al. Fraction size and dose parameters related to the incidence of pericardial effusions. Int J Radiat Oncol Biol Phys 1998;40:155-61. [Crossref] [PubMed]
- Bovelli D, Plataniotis G, Roila F, et al. Cardiotoxicity of chemotherapeutic agents and radiotherapy-related heart disease: ESMO Clinical Practice Guidelines. Ann Oncol 2010;21:v277-82. [Crossref] [PubMed]
- Taunk NK, Haffty BG, Kostis JB, et al. Radiation-induced heart disease: pathologic ab-normalities and putative mechanisms. Front Oncol 2015;5:39. [Crossref] [PubMed]
- Cohn KE, Stewart JR, Fajardo LF, et al. Heart disease following radiation. Medicine (Bal-timore) 1967;46:281-98.
- Mill WB, Baglan RJ, Kurichety P, et al. Symptomatic radiation-induced pericarditis in Hodgkin's disease. Int J Radiat Oncol Biol Phys 1984;10:2061-5. [Crossref] [PubMed]
- McEniery PT, Dorosti K, Schiavone WA, et al. Clinical and angiographic features of coronary artery disease after chest irradiation. Am J Cardiol 1987;60:1020-4. [Crossref] [PubMed]
- Jones JM, Ribeiro GG. Mortality patterns over 34 years of breast cancer patients in a clinical trial of post-operative radiotherapy. Clin Radiol 1989;40:204-8. [Crossref] [PubMed]
- Cosset JM, Henry-Amar M, Pellae-Cosset B, et al. Pericarditis and myocardial infarctions after Hodgkin's disease therapy. Int J Radiat Oncol Biol Phys 1991;21:447-9. [Crossref] [PubMed]
- Orzan F, Brusca A, Conte MR, et al. Severe coronary artery disease after radiation therapy of the chest and mediastinum: clinical presentation and treatment. Br Heart J 1993;69:496-500. [Crossref] [PubMed]
- Atkins KM, Rawal B, Chaunzwa TL, et al. Cardiac Radiation Dose, Cardiac Disease, and Mortality in Patients With Lung Cancer. J Am Coll Cardiol 2019;73:2976-87. [Crossref] [PubMed]
- Dorresteijn LD, Kappelle AC, Boogerd W, et al. Increased risk of ischemic stroke after radiotherapy on the neck in patients younger than 60 years. J Clin Oncol 2002;20:282-8. [Crossref] [PubMed]
- Wang X, Palaskas NL, Yusuf SW, et al. Incidence and Onset of Severe Cardiac Events After Radiotherapy for Esophageal Cancer. J Thorac Oncol 2020;15:1682-90. [Crossref] [PubMed]
- Banfill K, Giuliani M, Aznar M, et al. Cardiac Toxicity of Thoracic Radiotherapy: Existing Evidence and Future Directions. J Thorac Oncol 2021;16:216-27. [Crossref] [PubMed]
- Islam KM, Jiang X, Anggondowati T, et al. Comorbidity and Survival in Lung Cancer Patients. Cancer Epidemiol Biomarkers Prev 2015;24:1079-85. [Crossref] [PubMed]
- Janssen-Heijnen ML, Schipper RM, Razenberg PP, et al. Prevalence of co-morbidity in lung cancer patients and its relationship with treatment: a population-based study. Lung Cancer 1998;21:105-13. [Crossref] [PubMed]
- Bray F, Ferlay J, Soerjomataram I, et al. Global cancer statistics 2018: GLOBOCAN estimates of incidence and mortality worldwide for 36 cancers in 185 countries. CA Cancer J Clin 2018;68:394-424. [Crossref] [PubMed]
- Bradley JD, Paulus R, Komaki R, et al. Standard-dose versus high-dose conformal radiotherapy with concurrent and consolidation carboplatin plus paclitaxel with or without cetuximab for patients with stage IIIA or IIIB non-small-cell lung cancer (RTOG 0617): a randomised, two-by-two factorial phase 3 study. Lancet Oncol 2015;16:187-99. [Crossref] [PubMed]
- Sardaro A, Petruzzelli MF, D'Errico MP, et al. Radiation-induced cardiac damage in early left breast cancer patients: risk factors, biological mechanisms, radiobiology, and dosimetric constraints. Radiother Oncol 2012;103:133-42. [Crossref] [PubMed]
- Darby SC, McGale P, Taylor CW, et al. Long-term mortality from heart disease and lung cancer after radiotherapy for early breast cancer: prospective cohort study of about 300,000 women in US SEER cancer registries. Lancet Oncol 2005;6:557-65. [Crossref] [PubMed]
- Merzenich H, Baaken D, Schmidt M, et al. Cardiac late effects after modern 3D-conformal radiotherapy in breast cancer patients: a retrospective cohort study in Germany (ESCaRa). Breast Cancer Res Treat 2022;191:147-57. [Crossref] [PubMed]
- Hancock SL, Tucker MA, Hoppe RT. Factors affecting late mortality from heart disease after treatment of Hodgkin's disease. JAMA 1993;270:1949-55. [Crossref] [PubMed]
- van Nimwegen FA, Schaapveld M, Janus CP, et al. Cardiovascular disease after Hodgkin lymphoma treatment: 40-year disease risk. JAMA Intern Med 2015;175:1007-17. [Crossref] [PubMed]
- Autsavapromporn N, de Toledo SM, Little JB, et al. The role of gap junction communication and oxidative stress in the propagation of toxic effects among high-dose α-particle-irradiated human cells. Radiat Res 2011;175:347-57. [Crossref] [PubMed]
- Wang H, Wei J, Zheng Q, et al. Radiation-induced heart disease: a review of classification, mechanism and prevention. Int J Biol Sci 2019;15:2128-38. [Crossref] [PubMed]
- Yang EH, Marmagkiolis K, Balanescu DV, et al. Radiation-Induced Vascular Disease-A State-of-the-Art Review. Front Cardiovasc Med 2021;8:652761. [Crossref] [PubMed]
- Muroya Y, Plante I, Azzam EI, et al. High-LET ion radiolysis of water: visualization of the formation and evolution of ion tracks and relevance to the radiation-induced bystander effect. Radiat Res 2006;165:485-91. [Crossref] [PubMed]
- Hellweg CE. The Nuclear Factor κB pathway: A link to the immune system in the radiation response. Cancer Lett 2015;368:275-89. [Crossref] [PubMed]
- Ping Z, Peng Y, Lang H, et al. Oxidative Stress in Radiation-Induced Cardiotoxicity. Oxid Med Cell Longev 2020;2020:3579143. [Crossref] [PubMed]
- Halle M, Gabrielsen A, Paulsson-Berne G, et al. Sustained inflammation due to nuclear factor-kappa B activation in irradiated human arteries. J Am Coll Cardiol 2010;55:1227-36. [Crossref] [PubMed]
- Sievert W, Trott KR, Azimzadeh O, et al. Late proliferating and inflammatory effects on murine microvascular heart and lung endothelial cells after irradiation. Radiother Oncol 2015;117:376-81. [Crossref] [PubMed]
- Lorimore SA, Wright EG. Radiation-induced genomic instability and bystander effects: related inflammatory-type responses to radiation-induced stress and injury? A review. Int J Radiat Biol 2003;79:15-25. [Crossref] [PubMed]
- Chai Y, Lam RK, Calaf GM, et al. Radiation-induced non-targeted response in vivo: role of the TGFβ-TGFBR1-COX-2 signalling pathway. Br J Cancer 2013;108:1106-12. [Crossref] [PubMed]
- Koturbash I, Loree J, Kutanzi K, et al. In vivo bystander effect: cranial X-irradiation leads to elevated DNA damage, altered cellular proliferation and apoptosis, and increased p53 levels in shielded spleen. Int J Radiat Oncol Biol Phys 2008;70:554-62. [Crossref] [PubMed]
- Koturbash I, Rugo RE, Hendricks CA, et al. Irradiation induces DNA damage and modulates epigenetic effectors in distant bystander tissue in vivo. Oncogene 2006;25:4267-75. [Crossref] [PubMed]
- Baselet B, Sonveaux P, Baatout S, et al. Pathological effects of ionizing radiation: endothelial activation and dysfunction. Cell Mol Life Sci 2019;76:699-728. [Crossref] [PubMed]
- Citrin D, Cotrim AP, Hyodo F, et al. Radioprotectors and mitigators of radiation-induced normal tissue injury. Oncologist 2010;15:360-71. [Crossref] [PubMed]
- Nielsen KM, Offersen BV, Nielsen HM, et al. Short and long term radiation induced cardiovascular disease in patients with cancer. Clin Cardiol 2017;40:255-61. [Crossref] [PubMed]
- Olcina MM, Giaccia AJ. Reducing radiation-induced gastrointestinal toxicity - the role of the PHD/HIF axis. J Clin Invest 2016;126:3708-15. [Crossref] [PubMed]
- Stewart FA, Hoving S, Russell NS. Vascular damage as an underlying mechanism of cardiac and cerebral toxicity in irradiated cancer patients. Radiat Res 2010;174:865-9. [Crossref] [PubMed]
- Maier P, Hartmann L, Wenz F, et al. Cellular Pathways in Response to Ionizing Radiation and Their Targetability for Tumor Radiosensitization. Int J Mol Sci 2016;17:102. [Crossref] [PubMed]
- Panganiban RA, Day RM. Inhibition of IGF-1R prevents ionizing radiation-induced primary endothelial cell senescence. PLoS One 2013;8:e78589. [Crossref] [PubMed]
- Panganiban RA, Mungunsukh O, Day RM. X-irradiation induces ER stress, apoptosis, and senescence in pulmonary artery endothelial cells. Int J Radiat Biol 2013;89:656-67. [Crossref] [PubMed]
- Meeren AV, Bertho JM, Vandamme M, et al. Ionizing radiation enhances IL-6 and IL-8 production by human endothelial cells. Mediators Inflamm 1997;6:185-93. [Crossref] [PubMed]
- Azzam EI, Jay-Gerin JP, Pain D. Ionizing radiation-induced metabolic oxidative stress and prolonged cell injury. Cancer Lett 2012;327:48-60. [Crossref] [PubMed]
- Barnett GC, West CM, Dunning AM, et al. Normal tissue reactions to radiotherapy: towards tailoring treatment dose by genotype. Nat Rev Cancer 2009;9:134-42. [Crossref] [PubMed]
- Yarnold J, Brotons MC. Pathogenetic mechanisms in radiation fibrosis. Radiother Oncol 2010;97:149-61. [Crossref] [PubMed]
- Groarke JD, Nguyen PL, Nohria A, et al. Cardiovascular complications of radiation therapy for thoracic malignancies: the role for non-invasive imaging for detection of cardiovascular disease. Eur Heart J 2014;35:612-23. [Crossref] [PubMed]
- Gomm SA, Stretton TB. Chronic pericardial effusion after mediastinal radiotherapy. Thorax 1981;36:149-50. [Crossref] [PubMed]
- Marinko T. Pericardial disease after breast cancer radiotherapy. Radiol Oncol 2018;53:1-5. [Crossref] [PubMed]
- Basavaraju SR, Easterly CE. Pathophysiological effects of radiation on atherosclerosis development and progression, and the incidence of cardiovascular complications. Med Phys 2002;29:2391-403. [Crossref] [PubMed]
- Hufnagle JJ, Andersen SN, Maani EV. Radiation Therapy Induced Cardiac Toxicity. StatPearls. Treasure Island (FL): StatPearls Publishing, 2021.
- Lauk S, Trott KR. Endothelial cell proliferation in the rat heart following local heart irradiation. Int J Radiat Biol 1990;57:1017-30. [Crossref] [PubMed]
- Tamamori-Adachi M, Ito H, Sumrejkanchanakij P, et al. Critical role of cyclin D1 nuclear import in cardiomyocyte proliferation. Circ Res 2003;92:e12-9. [Crossref] [PubMed]
- Mele D, Rizzo P, Pollina AV, et al. Cancer therapy-induced cardiotoxicity: role of ultrasound deformation imaging as an aid to early diagnosis. Ultrasound Med Biol 2015;41:627-43. [Crossref] [PubMed]
- Altena R, Perik PJ, van Veldhuisen DJ, et al. Cardiovascular toxicity caused by cancer treatment: strategies for early detection. Lancet Oncol 2009;10:391-9. [Crossref] [PubMed]
- Galderisi M, Marra F, Esposito R, et al. Cancer therapy and cardiotoxicity: the need of serial Doppler echocardiography. Cardiovasc Ultrasound 2007;5:4. [Crossref] [PubMed]
- Lancellotti P, Nkomo VT, Badano LP, et al. Expert consensus for multi-modality imaging evaluation of cardiovascular complications of radiotherapy in adults: a report from the European Association of Cardiovascular Imaging and the American Society of Echocardiography. Eur Heart J Cardiovasc Imaging 2013;14:721-40. [Crossref] [PubMed]
- Tuohinen SS, Skyttä T, Poutanen T, et al. Radiotherapy-induced global and regional differences in early-stage left-sided versus right-sided breast cancer patients: speckle tracking echocardiography study. Int J Cardiovasc Imaging 2017;33:463-72. [Crossref] [PubMed]
- Walker V, Lairez O, Fondard O, et al. Early detection of subclinical left ventricular dysfunction after breast cancer radiation therapy using speckle-tracking echocardiography: association between cardiac exposure and longitudinal strain reduction (BACCARAT study). Radiat Oncol 2019;14:204. [Crossref] [PubMed]
- Thavendiranathan P, Poulin F, Lim KD, et al. Use of myocardial strain imaging by echocardiography for the early detection of cardiotoxicity in patients during and after cancer chemotherapy: a systematic review. J Am Coll Cardiol 2014;63:2751-68. [Crossref] [PubMed]
- Danad I, Raijmakers PG, Driessen RS, et al. Comparison of Coronary CT Angiography, SPECT, PET, and Hybrid Imaging for Diagnosis of Ischemic Heart Disease Determined by Fractional Flow Reserve. JAMA Cardiol 2017;2:1100-7. [Crossref] [PubMed]
- Abbott BG, Case JA, Dorbala S, et al. Contemporary Cardiac SPECT Imaging-Innovations and Best Practices: An Information Statement from the American Society of Nuclear Cardiology. J Nucl Cardiol 2018;25:1847-60. [Crossref] [PubMed]
- Díaz-Gavela AA, Figueiras-Graillet L, Luis ÁM, et al. Breast Radiotherapy-Related Cardiotoxicity. When, How, Why. Risk Prevention and Control Strategies. Cancers (Basel) 2021;13:1712. [Crossref] [PubMed]
- Landau D, Adams EJ, Webb S, et al. Cardiac avoidance in breast radiotherapy: a comparison of simple shielding techniques with intensity-modulated radiotherapy. Radiother Oncol 2001;60:247-55. [Crossref] [PubMed]
- Zeverino M, Petersson K, Kyroudi A, et al. A treatment planning comparison of contemporary photon-based radiation techniques for breast cancer. Phys Imaging Radiat Oncol 2018;7:32-8. [Crossref] [PubMed]
- Bergom C, Currey A, Desai N, et al. Deep Inspiration Breath Hold: Techniques and Advantages for Cardiac Sparing During Breast Cancer Irradiation. Front Oncol 2018;8:87. [Crossref] [PubMed]
- Curigliano G, Cardinale D, Dent S, et al. Cardiotoxicity of anticancer treatments: Epidemiology, detection, and management. CA Cancer J Clin 2016;66:309-25. [Crossref] [PubMed]
- Karpf D, Sakka M, Metzger M, et al. Left breast irradiation with tangential intensity modulated radiotherapy (t-IMRT) versus tangential volumetric modulated arc therapy (t-VMAT): trade-offs between secondary cancer induction risk and optimal target coverage. Radiat Oncol 2019;14:156. [Crossref] [PubMed]
- Pham TT, Ward R, Latty D, et al. Left-sided breast cancer loco-regional radiotherapy with deep inspiration breath-hold: Does volumetric-modulated arc radiotherapy reduce heart dose further compared with tangential intensity-modulated radiotherapy? J Med Imaging Radiat Oncol 2016;60:545-53. [Crossref] [PubMed]
- Corradini S, Ballhausen H, Weingandt H, et al. Left-sided breast cancer and risks of secondary lung cancer and ischemic heart disease : Effects of modern radiotherapy techniques. Strahlenther Onkol 2018;194:196-205. [Crossref] [PubMed]
- Sakka M, Kunzelmann L, Metzger M, et al. Cardiac dose-sparing effects of deep-inspiration breath-hold in left breast irradiation : Is IMRT more beneficial than VMAT? Strahlenther Onkol 2017;193:800-11. [Crossref] [PubMed]
- Maraldo MV, Brodin NP, Aznar MC, et al. Estimated risk of cardiovascular disease and secondary cancers with modern highly conformal radiotherapy for early-stage mediastinal Hodgkin lymphoma. Ann Oncol 2013;24:2113-8. [Crossref] [PubMed]
- Everett AS, Hoppe BS, Louis D, et al. Comparison of Techniques for Involved-Site Radiation Therapy in Patients With Lower Mediastinal Lymphoma. Pract Radiat Oncol 2019;9:426-34. [Crossref] [PubMed]
- Atkins KM, Bitterman DS, Chaunzwa TL, et al. Mean Heart Dose Is an Inadequate Surrogate for Left Anterior Descending Coronary Artery Dose and the Risk of Major Adverse Cardiac Events in Lung Cancer Radiation Therapy. Int J Radiat Oncol Biol Phys 2021;110:1473-9. [Crossref] [PubMed]
Cite this article as: Liu DW, Fu YR, Liu SH, Chen MYC, Hsu RJ, Hsu WL. The risk of cardiovascular toxicity caused by cancer radiotherapy—a narrative review. Ther Radiol Oncol 2022;6:4.