Imaging modalities to detect and track cancer treatment-related cardiovascular dysfunction
Introduction
Cardio-oncology is a new research field which focuses on cardiovascular (CV) disease associated with patients undergoing chemotherapy or radiation and cancer survivors. Cardio-oncology is also an important health issue in Taiwan, as the two leading causes of death are cancer and CV disease (CVD) (1). Cancer treatment has advanced rapidly from cytotoxic chemotherapy, radiation therapy (RT) and surgery to include targeted and immune-based therapies over the past decades (2). These advances in therapy have led to an increase in the number of cancer survivors (3), however an emerging issue associated with these new cancer therapies is side effects on the CV system, which cause different spectrums of morbidity and mortality (4). Cardiotoxicity refers to the direct harmful effects of cancer treatments on the CV system and/or the acceleration of CVD in addition to traditional CV risk factors (4,5). In order to detect cancer therapeutics-related cardiovascular dysfunction (CTRCD) as early as possible and mitigate the progression of CTRCD, it is important to be aware of the current guideline-recommended and promising imaging modalities. Therefore, in this study, we review these imaging modalities.
Evolving concepts of cardiotoxicity
Previous studies on cardiotoxicity have focused on anthracyclines and trastuzumab (6,7). Type I irreversible CTRCD, doxorubicin is the most well-known characteristic agent, is cumulative, dose-dependent and progressive while type II reversible CTRCD trastuzumab is the characteristic agent, is not cumulative, dose-dependent or progressive (8). However, recent arguments have arisen owing to doxorubicin-induced cardiotoxicity is not always irreversible (9,10) and trastuzumab-induced cardiotoxicity is not always reversible (11). Indeed, in addition to myocardial dysfunction, CTRCD should include all kinds of toxic/side effects affecting the CV system, including hypertension, endothelial and vascular dysfunction, accelerated atherosclerosis, thrombosis and bleeding, pulmonary hypertension, pericardial disease, QT prolongation, conduction disease/arrhythmias, as well as radiation-induced CVD (5,12-17). In addition, RT may cause damage to every component of the heart, which can present in the acute (<6 months) or late (3–30 years) phase (18,19). Breast cancer patients with RT have been reported to have greater risk of coronary heart disease and cardiac death compared to those without RT (20). The risk of CV events is higher in patients receiving RT concomitantly with anthracyclines (21,22). Patients with left‐sided breast cancer have a 1.4-fold higher risk of heart injury than those with right‐sided breast cancer (23). The rate of major adverse cardiac events (MACEs) has been shown to increase linearly by 7.4% per Gray (Gy) increase in mean heart dose (24). Therefore, it is important to consider which imaging modalities can be used to detect, diagnose and differentially diagnosis the increasingly varied types of CTRCD.
Imaging modalities for detecting cancer treatment-related cardiovascular dysfunction
Cancer therapy-related systolic myocardial dysfunction
For patients with symptoms or signs of current cardiac dysfunction, the guidelines recommend further assessing the risk using biomarkers such as troponins, natriuretic peptides, and evaluations of left ventricular (LV) ejection fraction (EF) (4). In addition to biomarkers, echocardiography‐based strain imaging may be particularly useful, and is recommended in guidelines as a baseline screening and follow-up imaging tool (25,26). A relative reduction in global longitudinal strain (GLS) of >15% from baseline is generally considered to be abnormal and an early sign of LV subclinical dysfunction (4) and an early indicator of heart failure. Cardiotoxicity of the right ventricle (RV) as identified by RV GLS follows a similar pattern to LV GLS (27). The left atrium (LA) acts as a reservoir for returning blood from pulmonary veins, and then releases blood to the LV during early diastole and contracts as a pump in late diastole. Therefore, left atrial strain (LAS) may be better able to evaluate diastolic function (28), and it has been strongly correlated with invasive measurements of LV end-diastolic pressure (wedge pressure) (29). Although trans-thoracic echocardiography (TTE) usually serves as the first-line imaging modalities in the evaluation of cardiac disease owing to its low cost, portability, widespread availability, lack of ionizing radiation, and ability to evaluate both anatomy and function of the heart, there are some conditions affecting measurements and accuracies causing limitations including patient’s position, patient’s condition (for example, lung hyperinflation, cutaneous emphysema, trauma, wound), effects of mechanical ventilation, suboptimal ultrasound windows (poor image quality), Heart plane motion during measurements, Doppler angle error (poor angle alignment) and arrhythmias (30). Dyspnea, often due to elevated LV filling pressure (LVFP), is a presenting symptom for patients with heart failure, and TEE provides a good algorithm for assessments of increasing LVFP with following parameters—peak tricuspid regurgitation velocity >2.8 m/s, E/e’ >14 and left atrial maximal volume index >34 mL/m2 (31,32). Aside from strain in echocardiography, cardiac magnetic resonance (CMR) imaging with T1 and T2 mapping may be particularly useful to evaluate CTRCD (26). The novel fast strain encoded CMR imaging (fast-SENC) also provides a highly reproducible method for assessing LV functional performance which is not dependent of the echogenic windows (33) and the ability of CMR strain has also been validated for the detection of cardiotoxicity and for the guidance of cardioprotective therapies (34). Low myocardial 18F-fluorodeoxyglucose (FDG) uptake before doxorubicin chemotherapy in heart positron emission tomography (PET) in patients with Hodgkin disease has been shown to predict the development of CTRCD (35). In addition, doxorubicin was associated with a dose-dependent increase in LV metabolic rate of glucose, particularly in the presence of low baseline FDG uptake in a mice model (35). This increase in myocardial FDG uptake has also been reported in patients following irradiation therapy (36). In addition, the presence or increase in mean standardized uptake value (SUV) of FDG uptake in the RV after anthracycline or trastuzumab treatment has been associated with cardiotoxicity in breast cancer patients (37). Figure 1 shows the summary of recommended imaging evaluations for cancer therapy-related systolic myocardial dysfunction.
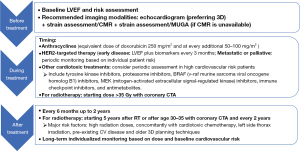
Cancer therapy-related atherosclerotic coronary and peripheral artery disease (PAD)
In addition to traditional CV risk factors, atherosclerotic processes can be accelerated by chemotherapy, RT and immune checkpoint inhibitor (ICI) in patients with cancer (38). Aside from traditional treadmill and vasodilator stress single photon emission tomography (SPECT) (39), stress echocardiography (38), stress CMR (40), computed tomography angiography (CTA) (41) and PET (39) are alternative evaluation tools for ischemia in patients receiving therapies that may cause vasospasms or accelerate atherosclerosis (26).
The time and frequency of follow-up depends on cancer treatment, cumulative dose of anthracyclines, dosing schedule and duration, and personal baseline CV risk (4). However, assessing myocardial viability is important for coronary interventionists to consider which vessels to treat to improve LV function, and stress echocardiography, SPECT (which uses perfusion tracers, thallium-201 or technetium-99m-sestamibi or -tetrofosmin, to assess both perfusion and cellular integrity, which in turn reflects tissue viability), PET (active glucose utilization as measured by PET with FDG) as well as CMR can be used for this purpose (42-44). In addition to RT (45), well-known chemotherapeutic agents that influence vascular function including vascular endothelial growth factor inhibitors (VEGFIs), tyrosine kinase inhibitors (TKIs), cisplatin, 5-fluorouracil, proteasome inhibitors (bortezomib and carfilzomib), immunomodulatory agents (thalidomide, lenalidomide and pomalidomide) (46) and even anthracyclines (47) have been reported to increase arterial stiffness, rate of PAD and stroke. Peripheral CTA can be used to quickly and accurately diagnose PAD after vascular claudication symptoms are reported in history taking with a positive and borderline ankle-brachial index (ABI) (41). However, 70% to 90% of people with an ABI value less than 0.90 either report no exertional leg symptoms (i.e., asymptomatic) or leg symptoms with walking that are not consistent with classic claudication (48). Although arterial duplex ultrasonography is a complementary modality that can help confirm the diagnosis, evaluate the extent of disease, monitor progression, and identify complications, the performer needs a sufficient understanding of the normal anatomy and caliber of arterial vessels, pathophysiology of arterial disease, and the hemodynamics of normal and abnormal flow as well as a baseline knowledge of the various ultrasonographic modalities and appropriate settings and techniques (49). Therefore, periodically surveying ABI is suggested in patients with RT and some chemotherapeutic agents.
Immunotherapy-related myocarditis
With the rapid advances in immunotherapies, more immunotherapy-related cardiotoxicities have been reported, with the current focus being on chimeric antigen receptor T cell (CAR-T) therapy-associated cytokine release syndrome (50) and ICI-associated myocarditis. Although the reported incidence of ICI‐related myocarditis is low (0.04–1.14%), it is associated with a high mortality rate (25–50%) and has been reported to occur early after the initiation of therapy (12,51-54). Although the diagnosis of myocarditis is challenging, elevated troponin and abnormal ECG findings are common (12,55). In addition, the prolonged use of ICIs has recently been linked to early progression of atherosclerosis, myocardial infarction and stroke (56,57).
In patients with myocardial edema, CMR with late gadolinium enhancement (LGE; which is often used to identify fibrosis) may be useful for an early diagnosis, however it has been reported to be present in <50% of those with ICI‐associated myocarditis (55,58). In addition, Zhang et al. (58) reported that elevated T2-weighted short tau inversion recovery (STIR), which often represents edema, was present in <30% overall. In their study, the presence of LGE, an LGE pattern, or elevated T2-weighted STIR were not associated with MACEs, however their study only included 103 subjects. Therefore, a larger, double-blinded, prospective study is needed to explore the causal relationship between LGE and/or elevated T2-weighted STIR and MACEs. Endomyocardial biopsy is the gold standard to diagnose ICI‐associated myocarditis, but it is often underused due to its invasive nature, risk of complications, and a lack of expertise in many hospitals (55,59). Therefore, CMR is an alternative approach to survey ICI-related myopathy.
Venous thromboembolism (VTE)
Patients with cancer are at a 4- to 7-fold higher risk of initial VTE, a 3-fold higher risk of recurrent VTE, a 2-fold higher risk of anticoagulation‐associated bleeding, and a 10‐fold higher risk of VTE-related death compared to patients without cancer (60). Similar to PAD, RT and chemotherapeutic agents including VEGFIs, TKIs, cisplatin, 5-fluorouracil, proteasome inhibitors, immunomodulatory agents (46) and even anthracyclines (47) have also been reported to increase the rate of VTE. In addition to treatment-related risk factors for VTE, cancer-related factors (e.g., primary site, histology and initial period after diagnosis), patient-related factors (e.g., age, ethnicity and comorbidities) and some biomarkers (e.g., platelet count, white count, hemoglobin, tissue factor, D-dimer, P-selectin and thrombin generation potential) should also be taken into consideration (61). Clinically suspected VTE [including pulmonary embolism (PE) and deep vein thrombosis (DVT)] have become the most common indications for CTA in emergency departments (62). However, CTA can diagnose PE of major vessels but is not sufficiently sensitive to exclude PE due to its poor sensitivity for subsegmental pulmonary vessels, and ventilation/perfusion (V/Q) lung scans are better for subsegmental pulmonary vessels (63). Compression ultrasonography is also a good alternative to detect DVT in emergency departments (63). Figure 2 shows the summary of recommended risk assessments and imaging evaluations for cancer-related VTE.
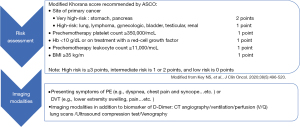
Pericardial disease and utility of cardiac imaging
Pericardial disease from effusion, tamponade to constrictive pericarditis can be occurred owing to cancer per se (primary or through metastasis) or cancer treatment (chemotherapy and radiotherapy) (64). Echocardiography is the first and often the only necessary imaging test in patients with acute pericarditis and is essential to identify complications, such as tamponade or constrictive pericarditis, and could be useful for monitoring the evolution of pericardial effusion over time and the response to medical therapy (65). While CMR is usually applied for tissue characterisation, CT scan image tumour invasion of adjacent structures which guides the surgeon for tumor operative removal. PET scanning can be very helpful in determining an active or remitted lesion (64).
Conclusions
Since CTRCD should include all kinds of toxic/side effects affecting the whole CV system, multiple imaging modalities are needed to make a differential diagnosis. Proper imaging monitoring protocols at baseline, subsequent follow-up, and suggested management in patients with suspected CTRCD are important to detect as early as possible and prevent the deterioration of CTRCD with proper management in cancer patients receiving cancer therapies.
Acknowledgments
Funding: This study was partly supported by the Ministry of Science and Technology of Taiwan (108-2314-B-418-002-MY3, 110-2314-B-418-005-MY3).
Footnote
Provenance and Peer Review: The article was commissioned by the editorial office, Therapeutic Radiology and Oncology for the series “Cardio-Oncology”. The article has undergone external peer review.
Conflicts of Interest: All authors have completed the ICMJE uniform disclosure form (available at https://dx.doi.org/10.21037/tro-21-17). The series “Cardio-Oncology” was commissioned by the editorial office without any funding or sponsorship. YWW served as an unpaid Guest Editor for the series. The authors have no other conflicts of interest to declare.
Ethical Statement: The authors are accountable for all aspects of the work in ensuring that questions related to the accuracy or integrity of any part of the work are appropriately investigated and resolved.
Open Access Statement: This is an Open Access article distributed in accordance with the Creative Commons Attribution-NonCommercial-NoDerivs 4.0 International License (CC BY-NC-ND 4.0), which permits the non-commercial replication and distribution of the article with the strict proviso that no changes or edits are made and the original work is properly cited (including links to both the formal publication through the relevant DOI and the license). See: https://creativecommons.org/licenses/by-nc-nd/4.0/.
References
- Taiwan Ministry of Health and Welfare. Cause of Death Statistics 2020.
- McCune JS. Rapid Advances in Immunotherapy to Treat Cancer. Clin Pharmacol Ther 2018;103:540-4. [Crossref] [PubMed]
- Siegel RL, Miller KD, Jemal A. Cancer statistics, 2019. CA Cancer J Clin 2019;69:7-34. [Crossref] [PubMed]
- Zamorano JL, Lancellotti P, Rodriguez Muñoz D, et al. 2016 ESC Position Paper on cancer treatments and cardiovascular toxicity developed under the auspices of the ESC Committee for Practice Guidelines: The Task Force for cancer treatments and cardiovascular toxicity of the European Society of Cardiology (ESC). Eur Heart J 2016;37:2768-801. [Crossref] [PubMed]
- Armstrong GT, Oeffinger KC, Chen Y, et al. Modifiable risk factors and major cardiac events among adult survivors of childhood cancer. J Clin Oncol 2013;31:3673-80. [Crossref] [PubMed]
- Ewer MS, Lippman SM. Type II chemotherapy-related cardiac dysfunction: time to recognize a new entity. J Clin Oncol 2005;23:2900-2. [Crossref] [PubMed]
- Wang CC, Wu CK, Tsai ML, et al. 2019 Focused Update of the Guidelines of the Taiwan Society of Cardiology for the Diagnosis and Treatment of Heart Failure. Acta Cardiol Sin 2019;35:244-83. [PubMed]
- Plana JC, Galderisi M, Barac A, et al. Expert consensus for multimodality imaging evaluation of adult patients during and after cancer therapy: a report from the American Society of Echocardiography and the European Association of Cardiovascular Imaging. Eur Heart J Cardiovasc Imaging 2014;15:1063-93. [Crossref] [PubMed]
- Cardinale D, Colombo A, Lamantia G, et al. Anthracycline-induced cardiomyopathy: clinical relevance and response to pharmacologic therapy. J Am Coll Cardiol 2010;55:213-20. [Crossref] [PubMed]
- Cardinale D, Colombo A, Bacchiani G, et al. Early detection of anthracycline cardiotoxicity and improvement with heart failure therapy. Circulation 2015;131:1981-8. [Crossref] [PubMed]
- de Azambuja E, Procter MJ, van Veldhuisen DJ, et al. Trastuzumab-associated cardiac events at 8 years of median follow-up in the Herceptin Adjuvant trial (BIG 1-01). J Clin Oncol 2014;32:2159-65. [Crossref] [PubMed]
- Mahmood SS, Fradley MG, Cohen JV, et al. Myocarditis in Patients Treated With Immune Checkpoint Inhibitors. J Am Coll Cardiol 2018;71:1755-64. [Crossref] [PubMed]
- Bates JE, Howell RM, Liu Q, et al. Therapy-Related Cardiac Risk in Childhood Cancer Survivors: An Analysis of the Childhood Cancer Survivor Study. J Clin Oncol 2019;37:1090-101. [Crossref] [PubMed]
- Henry ML, Niu J, Zhang N, et al. Cardiotoxicity and Cardiac Monitoring Among Chemotherapy-Treated Breast Cancer Patients. JACC Cardiovasc Imaging 2018;11:1084-93. [Crossref] [PubMed]
- Bijl JM, Roos MM, van Leeuwen-Segarceanu EM, et al. Assessment of Valvular Disorders in Survivors of Hodgkin's Lymphoma Treated by Mediastinal Radiotherapy ± Chemotherapy. Am J Cardiol 2016;117:691-6. [Crossref] [PubMed]
- Cheng KH, Handschumacher MD, Assuncao BMBL, et al. Contraction Timing Patterns in Patients Treated for Breast Cancer Before and After Anthracyclines Therapy. J Am Soc Echocardiogr 2017;30:454-60. [Crossref] [PubMed]
- Heidenreich PA, Hancock SL, Lee BK, et al. Asymptomatic cardiac disease following mediastinal irradiation. J Am Coll Cardiol 2003;42:743-9. [Crossref] [PubMed]
- Desai MY, Windecker S, Lancellotti P, et al. Prevention, Diagnosis, and Management of Radiation-Associated Cardiac Disease: JACC Scientific Expert Panel. J Am Coll Cardiol 2019;74:905-27. [Crossref] [PubMed]
- Nielsen KM, Offersen BV, Nielsen HM, et al. Short and long term radiation induced cardiovascular disease in patients with cancer. Clin Cardiol 2017;40:255-61. [Crossref] [PubMed]
- Cheng YJ, Nie XY, Ji CC, et al. Long-Term Cardiovascular Risk After Radiotherapy in Women With Breast Cancer. J Am Heart Assoc 2017;6:005633. [Crossref] [PubMed]
- Boekel NB, Jacobse JN, Schaapveld M, et al. Cardiovascular disease incidence after internal mammary chain irradiation and anthracycline-based chemotherapy for breast cancer. Br J Cancer 2018;119:408-18. [Crossref] [PubMed]
- Boekel NB, Duane FK, Jacobse JN, et al. Heart failure after treatment for breast cancer. Eur J Heart Fail 2020;22:366-74. [Crossref] [PubMed]
- Taylor C, McGale P, Brønnum D, et al. Cardiac Structure Injury After Radiotherapy for Breast Cancer: Cross-Sectional Study With Individual Patient Data. J Clin Oncol 2018;36:2288-96. [Crossref] [PubMed]
- Darby SC, Ewertz M, McGale P, et al. Risk of ischemic heart disease in women after radiotherapy for breast cancer. N Engl J Med 2013;368:987-98. [Crossref] [PubMed]
- Armenian SH, Lacchetti C, Lenihan D. Prevention and Monitoring of Cardiac Dysfunction in Survivors of Adult Cancers: American Society of Clinical Oncology Clinical Practice Guideline Summary. J Oncol Pract 2017;13:270-5. [Crossref] [PubMed]
- Plana JC, Thavendiranathan P, Bucciarelli-Ducci C, et al. Multi-Modality Imaging in the Assessment of Cardiovascular Toxicity in the Cancer Patient. JACC Cardiovasc Imaging 2018;11:1173-86. [Crossref] [PubMed]
- Keramida K, Farmakis D. Right ventricular involvement in cancer therapy-related cardiotoxicity: the emerging role of strain echocardiography. Heart Fail Rev 2021;26:1189-93. [Crossref] [PubMed]
- Morris DA, Belyavskiy E, Aravind-Kumar R, et al. Potential Usefulness and Clinical Relevance of Adding Left Atrial Strain to Left Atrial Volume Index in the Detection of Left Ventricular Diastolic Dysfunction. JACC Cardiovasc Imaging 2018;11:1405-15. [Crossref] [PubMed]
- Cameli M, Sparla S, Losito M, et al. Correlation of Left Atrial Strain and Doppler Measurements with Invasive Measurement of Left Ventricular End-Diastolic Pressure in Patients Stratified for Different Values of Ejection Fraction. Echocardiography 2016;33:398-405. [Crossref] [PubMed]
- Huang SJ, McLean AS. Appreciating the strengths and weaknesses of transthoracic echocardiography in hemodynamic assessments. Cardiol Res Pract 2012;2012:894308. [Crossref] [PubMed]
- Nagueh SF. Left Ventricular Diastolic Function: Understanding Pathophysiology, Diagnosis, and Prognosis With Echocardiography. JACC Cardiovasc Imaging 2020;13:228-44. [Crossref] [PubMed]
- Nagueh SF. Non-invasive assessment of left ventricular filling pressure. Eur J Heart Fail 2018;20:38-48. [Crossref] [PubMed]
- Giusca S, Korosoglou G, Zieschang V, et al. Reproducibility study on myocardial strain assessment using fast-SENC cardiac magnetic resonance imaging. Sci Rep 2018;8:14100. [Crossref] [PubMed]
- Giusca S, Korosoglou G, Montenbruck M, et al. Multiparametric Early Detection and Prediction of Cardiotoxicity Using Myocardial Strain, T1 and T2 Mapping, and Biochemical Markers: A Longitudinal Cardiac Resonance Imaging Study During 2 Years of Follow-Up. Circ Cardiovasc Imaging 2021;14:e012459. [Crossref] [PubMed]
- Bauckneht M, Ferrarazzo G, Fiz F, et al. Doxorubicin Effect on Myocardial Metabolism as a Prerequisite for Subsequent Development of Cardiac Toxicity: A Translational 18F-FDG PET/CT Observation. J Nucl Med 2017;58:1638-45. [Crossref] [PubMed]
- Zöphel K, Hölzel C, Dawel M, et al. PET/CT demonstrates increased myocardial FDG uptake following irradiation therapy. Eur J Nucl Med Mol Imaging 2007;34:1322-3. [Crossref] [PubMed]
- Kim J, Cho SG, Kang SR, et al. Association between FDG uptake in the right ventricular myocardium and cancer therapy-induced cardiotoxicity. J Nucl Cardiol 2020;27:2154-63. [Crossref] [PubMed]
- Novo G, Santoro C, Manno G, et al. Usefulness of Stress Echocardiography in the Management of Patients Treated with Anticancer Drugs. J Am Soc Echocardiogr 2021;34:107-16. [Crossref] [PubMed]
- Task Force Members. 2013 ESC guidelines on the management of stable coronary artery disease: the Task Force on the management of stable coronary artery disease of the European Society of Cardiology. Eur Heart J 2013;34:2949-3003. [Crossref] [PubMed]
- Burrage MK, Ferreira VM. The use of cardiovascular magnetic resonance as an early non-invasive biomarker for cardiotoxicity in cardio-oncology. Cardiovasc Diagn Ther 2020;10:610-24. [Crossref] [PubMed]
- Layoun ME, Yang EH, Herrmann J, et al. Applications of Cardiac Computed Tomography in the Cardio-Oncology Population. Curr Treat Options Oncol 2019;20:47. [Crossref] [PubMed]
- Di Carli MF, Maddahi J, Rokhsar S, et al. Long-term survival of patients with coronary artery disease and left ventricular dysfunction: implications for the role of myocardial viability assessment in management decisions. J Thorac Cardiovasc Surg 1998;116:997-1004. [Crossref] [PubMed]
- Arnese M, Cornel JH, Salustri A, et al. Prediction of improvement of regional left ventricular function after surgical revascularization. A comparison of low-dose dobutamine echocardiography with 201Tl single-photon emission computed tomography. Circulation 1995;91:2748-52. [Crossref] [PubMed]
- Patel H, Mazur W, Williams KA Sr, et al. Myocardial viability-State of the art: Is it still relevant and how to best assess it with imaging? Trends Cardiovasc Med 2018;28:24-37. [Crossref] [PubMed]
- Yang EH, Marmagkiolis K, Balanescu DV, et al. Radiation-Induced Vascular Disease-A State-of-the-Art Review. Front Cardiovasc Med 2021;8:652761. [Crossref] [PubMed]
- Zito C, Manganaro R, Carerj S, et al. Peripheral Artery Disease and Stroke. J Cardiovasc Echogr 2020;30:S17-25. [Crossref] [PubMed]
- Parr SK, Liang J, Schadler KL, et al. Anticancer Therapy-Related Increases in Arterial Stiffness: A Systematic Review and Meta-Analysis. J Am Heart Assoc 2020;9:e015598. [Crossref] [PubMed]
- Polonsky TS, McDermott MM. Lower Extremity Peripheral Artery Disease Without Chronic Limb-Threatening Ischemia: A Review. JAMA 2021;325:2188-98. [Crossref] [PubMed]
- Nasra K, Osher M. Sonography Vascular Peripheral Arterial Assessment, Protocols, And Interpretation. StatPearls. Treasure Island, FL, USA: StatPearls Publishing, 2021.
- Alvi RM, Frigault MJ, Fradley MG, et al. Cardiovascular Events Among Adults Treated With Chimeric Antigen Receptor T-Cells (CAR-T). J Am Coll Cardiol 2019;74:3099-108. [Crossref] [PubMed]
- Salem JE, Manouchehri A, Moey M, et al. Cardiovascular toxicities associated with immune checkpoint inhibitors: an observational, retrospective, pharmacovigilance study. Lancet Oncol 2018;19:1579-89. [Crossref] [PubMed]
- Johnson DB, Balko JM, Compton ML, et al. Fulminant Myocarditis with Combination Immune Checkpoint Blockade. N Engl J Med 2016;375:1749-55. [Crossref] [PubMed]
- Moslehi JJ, Salem JE, Sosman JA, et al. Increased reporting of fatal immune checkpoint inhibitor-associated myocarditis. Lancet 2018;391:933. [Crossref] [PubMed]
- Ganatra S, Neilan TG. Immune Checkpoint Inhibitor-Associated Myocarditis. Oncologist 2018;23:879-86. [Crossref] [PubMed]
- Agrawal N, Khunger A, Vachhani P, et al. Cardiac Toxicity Associated with Immune Checkpoint Inhibitors: Case Series and Review of the Literature. Case Rep Oncol 2019;12:260-76. [Crossref] [PubMed]
- Drobni ZD, Alvi RM, Taron J, et al. Association Between Immune Checkpoint Inhibitors With Cardiovascular Events and Atherosclerotic Plaque. Circulation 2020;142:2299-311. [Crossref] [PubMed]
- Stein-Merlob AF, Rothberg MV, Ribas A, et al. Cardiotoxicities of novel cancer immunotherapies. Heart 2021;107:1694-703. [Crossref] [PubMed]
- Zhang L, Awadalla M, Mahmood SS, et al. Cardiovascular magnetic resonance in immune checkpoint inhibitor-associated myocarditis. Eur Heart J 2020;41:1733-43. [Crossref] [PubMed]
- Caforio AL, Pankuweit S, Arbustini E, et al. Current state of knowledge on aetiology, diagnosis, management, and therapy of myocarditis: a position statement of the European Society of Cardiology Working Group on Myocardial and Pericardial Diseases. Eur Heart J 2013;34:2636-48, 2648a-2648d.
- Streiff MB, Abutalib SA, Farge D, et al. Update on Guidelines for the Management of Cancer-Associated Thrombosis. Oncologist 2021;26:e24-40. [Crossref] [PubMed]
- Lyman GH. Venous thromboembolism in the patient with cancer: focus on burden of disease and benefits of thromboprophylaxis. Cancer 2011;117:1334-49. [Crossref] [PubMed]
- Douek P, Rotzinger DC, Meuli RA, et al. Impact of CT venography added to CT pulmonary angiography for the detection of deep venous thrombosis and relevant incidental CT findings. Eur J Radiol 2020;133:109388. [Crossref] [PubMed]
- Weg JG. Current diagnostic techniques for pulmonary embolism. Semin Vasc Surg 2000;13:182-8. [PubMed]
- Ghosh AK, Crake T, Manisty C, et al. Pericardial Disease in Cancer Patients. Curr Treat Options Cardiovasc Med 2018;20:60. [Crossref] [PubMed]
- Chiabrando JG, Bonaventura A, Vecchié A, et al. Management of Acute and Recurrent Pericarditis: JACC State-of-the-Art Review. J Am Coll Cardiol 2020;75:76-92. [Crossref] [PubMed]
Cite this article as: Cheng KH, Hou CJY, Hung CM, Wu YW. Imaging modalities to detect and track cancer treatment-related cardiovascular dysfunction. Ther Radiol Oncol 2021;5:23.