Feasibility assessment of proton treatment for ventricular tachycardia—treatment planning study
Introduction
Ventricular tachycardia (VT) is a common cause of sudden cardiac death (1). Traditional cardiology has several methods to treat patients with the disease, including drug therapy, catheter ablation and high voltage shock treatment (2). Implantable cardioverter-defibrillators (ICDs) positively affected survival in patients at increased risk for ventricular arrhythmias (3). Nevertheless, ICDs do not prevent ventricular arrhythmias. In the current era, the use of catheter ablation for VT is well supported in patients with recurrent VT refractory to anti-arrhythmic drugs (AADs) and in patients for whom AADs are poorly tolerated (4). Catheter ablation is not curative for many patients (5). Common reasons for catheter ablation include inaccessible arrhythmogenic tissue and an inability to delivery adequate ablative energy transmurally across ventricular myocardium (6).
Many studies have investigated the application of radioablation as an alternative method to treat patients with recurrent VT. By delivering high dose photon beams in one fraction, the treatment effect is similar to ablation in mitigating the arrhythmic burden (7-10). Radioablation has been demonstrated as an effective treatment option for patients suffering from VT (7). The workflow for radioablation is similar to that of stereotactic body radiation therapy (SBRT), with a conventional treatment dose deliver within 3 to 10 fractions (11,12). Due to the feather of rapid dose fall off and the high dose per fraction treatment, caution must be paid to the uncertainty of dose delivery, including respiratory and cardiac motions. Furthermore, the procedure from CT simulation to dosimetry verification, requires enhanced accuracy compared to that of conventional photon therapy.
The unique feature of the proton beam treatment lies in the rapid distal dose fall-off behind the Bragg peak, while proton treatment has been widely applied worldwide for the treatment of various cancers. Over the past 5 years approximately 3,000 patients have received proton treatment at Linkou Chang Gung Memorial Hospital Proton Center, and the further applications for benign diseases are currently being developed (13,14). Based on the experience, the feasibility of a single fraction proton irradiation to treat VT disease requires assessment (15). The present report aims to compare the effectiveness of the photon beam and proton beams in different subtracts where VT commonly occurs. We present the following article in accordance with the MDAR reporting checklist (available at https://tro.amegroups.com/article/view/10.21037/tro-21-43/rc).
Methods
Patient selection
Two patients with VT and an ICD were retrospective included in this study, who having had at least 3 episodes of ICD-treated VT within the preceding 3 months, and having undergone at least 1 catheter ablation procedure. The study was conducted in accordance with the Declaration of Helsinki (as revised in 2013). The retrospective study has been approved by Chang Gung Medical Foundation Institutional Review Board (No. 202200668B0) and individual consent for this retrospective analysis was waived.
Computed tomography (CT) simulation
The patient with three sets of CT were retrospective selected, including (I) a free breath three dimensional (3D) CT for treatment planning, (II) a free-breath CT with contrast for delineation of cardiac structure, (III) a four-dimensional (4D) CT for motion evaluation. The scanning range was defined as the target region in the superior-inferior direction for 10 cm to cover the non-coplanar treatment plan dose calculation.
The CT images were imported into the Eclipse (Varian Medical, Palo Alto, CA, USA) treatment planning software version 13.7 for target delineation and development of treatment plan.
Target delineation
For the photon treatment plan, the gross tumor volume (GTV), internal target volume (ITV) and planning target volume (PTV) were defined. The GTV was defined by the contouring of five common recurrent VT regions by cardiology physician, including apical anterior (AA), apical inferior (AI), basal septal (BS), inferior basal (IB), lateral basal (LB) (16-20). The five regions are shown in Figure 1, and were separated into 2 groups for organ at risk (OAR) dose analysis: AA and AI in Group 1; BS, IB and LB in Group 2, due the locations at the center or surface of the heart. The ITV were defined as the GTV expanded margins, including by respiratory and cardiac motions, with the combined effect being assessed by all the phases of the 4D-CT, overlaid with the reference CT. The PTV was defined as the ITV with a 5 mm expansion to compensate for setup and motion uncertainty. No clinical target volume (CTV) expansion was used for this study.
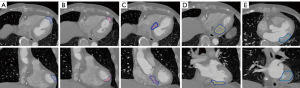
For the proton treatment plan, the definition of GTVs and ITVs were defined in the same manner as the photon treatment plan, but included independent longitudinal and lateral margins for the proton planning target volume (PPTV). The longitudinal margin considered range uncertainty (3.5% of range + 3 mm), while the lateral margin considered setup uncertainty, internal target motion and penumbra according treatment to site and field size and energy.
Nearby OAR including the lungs, esophagus, stomach and heart were contoured by a radiation oncologist on 3D CT images for dose evaluation.
Treatment planning
All treatment planning was conducted using a single fraction of 25 Gy was prescribed to the treatment target. For photon treatment planning, Varian EDGE (Varian Medical, Palo Alto, CA, USA) with 6X flattering filter free (FFF) were used in the study (21), as FFF reduces the treatment time by applying an beam intensity, while removal of the flattening filter reduces out-of-field dose. The dose rate was set as 1,400 MU/min. Four to five coplanar and non-coplanar partial arcs were used for volumetric modulated arc therapy (VMAT) plan with gantry angle of 30° to 330° and couch angles of 330° to 30° respectively. Progressive resolution optimizer (PRO) was used for photon treatment planning optimization, and analytical anisotropic algorithm (AAA) was used for dose calculating, while dose grids were set as 1 mm.
For the proton treatment planning, wobbling (WB) and single field optimization (SFO) of pencil beam scanning (PBS) treatment techniques both with five fields were used. The WB irradiates a uniform dose under a restricted block radius by rotating a pencil beam with x and y magnets and passing through a scatter, and spread-out Bragg peak (SOBP) are generated by ridge filter. PBS can perform intensity modulated dose distribution by varying the energy, position of each pencil beam. The beam angle selection was to minimize the beam overlapping region, while gantry angles of the non-coplanar were used to achieve a high gradient dose distribution. PCS_RF_13721 for wobbling technique dose calculation and non-linear proton optimizer (NUPO)_PB_13721 were used for SFO technique, and PCS_PB_13721 was used for dose calculation
Plan evaluation
The conformal index (CI), homogeneity index (HI) and R50 were used to evaluate the target dose (22-24). HI is the index to that can evaluate the homogeneity in PTV, and CI is to evaluate the region covered by the full prescribed dose between the different treatment plans. Dose in OAR were evaluated by using dose-volume histogram (DVH) and maximum dose.
where D5% and D95% are dose received by the 5% and 95% of the PTV respectively.
where VPTV is the volume of PTV, VPTVref is the volume of PTV receiving prescribe dose, an ideal plan ensuring perfect dose coverage would be 1.
R50, R30 are the ratio of the 50% and 30% of prescription isodose volume to the volume of PTV.
Others OAR including the heart (V5Gy to V25Gy, mean dose), lungs, esophagus, spinal cord, and left anterior descending artery (LAD) were used to evaluate photon and proton wobbling and pencil beam treatment plans. Note that V5 is defined as the percentage of the total normal heart that is irradiated with a dose of ≥5 Gy, V10Gy to V25Gy is defined in the same way.
Statistical analyses
The nonparametric test Mann Whitney U test was used to compare dosimetric parameters between Group 1 and Group 2, and WB and PBS. Spearman correlation coefficient was used to identify relations between the volume of CTV and the mean heart dose in the different treatment technique. Statistical analysis was performed using SPSS 24. All P values less than 0.05 were considered to be significant.
Results
Figure 1 shows the contouring of five GTV regions contoured by a cardiologist. The volume of five different subtract GTVs of all patients were 1.4 to 12 cm3, while CTVs were 6.33 to 53.67 cm3. Table 1 is the CI and HI under the same dose coverage. The CI of the photon VMAT plan and proton (WB and PBS) plans were 0.830±0.105, 0.946±0.076, respectively, with a P value <0.05. The HI of the photon and proton were 1.069±0.025, 1.048±0.018, respectively, with a P value <0.05. Additionally, Table 2 compares the CI and HI of the WB and PBS treatment planning, with P>0.05 with no difference in both results.
Table 1
Evaluation index | Photon (VMAT) | Proton (WB and PBS) | P value |
---|---|---|---|
CI | 0.830±0.105 | 0.946±0.076 | 0.0008* |
HI | 1.069±0.025 | 1.048±0.018 | 0.006* |
*, P<0.05. CI, conformal index; HI, homogeneity index; VMAT, photon volumetric modulated arc therapy; WB, proton wobbling; PBS, proton pencil beam scanning.
Table 2
Evaluation index | WB | PBS | P value |
---|---|---|---|
CI | 0.951±0.064 | 0.941±0.089 | 0.38 |
HI | 1.043±0.019 | 1.054±0.015 | 0.075 |
CI, conformal index; HI, homogeneity index; WB, proton wobbling; PBS, proton pencil beam scanning.
To evaluate the R50, R30 and dose to OAR, the five subtracts were divided into 2 groups: Group 1 and Group 2. As the locations of the heart and volume were different, the separation into 2 groups was performed in order to obtain more accurate results.
Tables 3,4 shows the R50 and R30 in Group 1 and Group 2 to compare the photon plan and proton treatment plans. In Group 1, the R50 and R30 were 4.19±0.58 and 7.32±1.08, respectively, in the photon plans, and 4.34±1.03 and 6.7±1.78, respectively in the proton plan, both with both P value >0.05. In Group 2, the R50 shows no difference between photon and proton plans. However, for R30, it shows 9.98±1.65 in the photon plans and 7.12±1.02 in the proton plans with P value <0.0001. Tables 5,6 compare the R50 and R30 in the WB and PBS plana, both the R50 and R30 of the PBS plan in Group 2 exhibit a difference, while there is no difference in Group 1.
Table 3
Dosimetric parameter | Photon (VMAT) | Proton (WB and PBS) | P value |
---|---|---|---|
R50 | 4.19±0.58 | 4.34±1.03 | 0.39 |
R30 | 7.32±1.08 | 6.7±1.78 | 0.28 |
R50, ratio of the 50% of prescription isodose volume to the volume of PTV; R30, ratio of the 30% of prescription isodose volume to the volume of PTV; VMAT, photon volumetric modulated arc therapy; WB, proton wobbling; PBS, proton pencil beam scanning.
Table 4
Dosimetric parameter | Photon (VMAT) | Proton (WB and PBS) | P value |
---|---|---|---|
R50 | 4.02±0.63 | 4.42±0.57 | 0.1 |
R30 | 9.98±1.65 | 7.12±1.02 | 0.0001* |
*, P<0.05. R50, ratio of the 50% of prescription isodose volume to the volume of PTV; R30, ratio of the 30% of prescription isodose volume to the volume of PTV; VMAT, photon volumetric modulated arc therapy; WB, proton wobbling; PBS, proton pencil beam scanning.
Table 5
Dosimetric parameter | WB | PBS | P value |
---|---|---|---|
R50 | 3.976±0.631 | 4.721±1.309 | 0.17 |
R30 | 5.644±1.086 | 6.92±2.262 | 0.17 |
R50, ratio of the 50% of prescription isodose volume to the volume of PTV; R30, ratio of the 30% of prescription isodose volume to the volume of PTV; WB, proton wobbling; PBS, proton pencil beam scanning.
Table 6
Dosimetric parameter | WB | PBS | P value |
---|---|---|---|
R50 | 4.74±0.455 | 4.112±0.518 | 0.024* |
R30 | 7.765±0.855 | 6.486±0.77 | 0.01* |
*, P<0.05. R50, ratio of the 50% of prescription isodose volume to the volume of PTV; R30, ratio of the 30% of prescription isodose volume to the volume of PTV; WB, proton wobbling; PBS, proton pencil beam scanning.
Tables 7,8 show doses to normal heart received in the photon and proton plans in Group 1 and Group 2. The results reveal the volume of the heart receiving the same dose was larger in the photon treatment plan than in the proton plan in most evaluations. But there was a significant difference between the photon and proton plans only in the volume upon receiving 5 and 10 Gy in both Group 1 and Group 2, and no difference in the volume upon receiving 15 to 25 Gy between the two groups. However, in the comparison of the WB and PBS plans of Group 1 and Group 2, as shown in Tables 9,10, the results revealed no difference.
Table 7
Dosimetric parameter | Photon VMAT | Proton (WB and PBS) | P value |
---|---|---|---|
V5Gy | 17.43±6.75 | 7.20±1.84 | 0.001* |
V10Gy | 8.72±2.70 | 5.53±1.44 | 0.01* |
V15Gy | 5.68±1.54 | 4.41±1.12 | 0.07 |
V20Gy | 3.83±0.84 | 3.42±0.88 | 0.23 |
V25Gy | 2.48±0.61 | 2.01±0.68 | 0.14 |
Mean dose | 3.48±0.91 | 1.37±0.35 | 0.00001* |
*, P<0.05. VMAT, photon volumetric modulated arc therapy; WB, proton wobbling; PBS, proton pencil beam scanning.
Table 8
Dosimetric parameter | Photon VMAT | Proton (WB and PBS) | P value |
---|---|---|---|
V5Gy | 40.85±13.77 | 28.42±9.58 | 0.02* |
V10Gy | 23.19±7.22 | 17.65±4.85 | 0.04* |
V15Gy | 13.47±3,81 | 13.28±2.99 | 0.45 |
V20Gy | 8.37±1.67 | 9.87±2.14 | 0.08 |
V25Gy | 5.00±0.86 | 5.52±1.24 | 0.19 |
Mean dose | 6.38±1.58 | 4.76±1.35 | 0.02* |
*, P<0.05. VMAT, photon volumetric modulated arc therapy; WB, proton wobbling; PBS, proton pencil beam scanning.
Table 9
Dosimetric parameter | WB | PBS | P value |
---|---|---|---|
V5Gy | 6.17±1.65 | 8.22±1.55 | 0.06 |
V10Gy | 4.76±1.39 | 6.30±1.15 | 0.06 |
V15Gy | 3.88±1.16 | 4.96±0.89 | 0.09 |
V20Gy | 3.06±0.98 | 3.78±0.71 | 0.14 |
V25Gy | 1.75±0.77 | 2.26±0.57 | 0.16 |
Mean dose | 1.18±0.34 | 1.57±0.27 | 0.06 |
WB, proton wobbling; PBS, proton pencil beam scanning.
Table 10
Dosimetric parameter | WB | PBS | P value |
---|---|---|---|
V5Gy | 30.12±10.42 | 26.73±9.29 | 0.28 |
V10Gy | 18.34±5.50 | 16.98±4.52 | 0.32 |
V15Gy | 13.79±3.46 | 12.78±2.67 | 0.29 |
V20Gy | 10.11±2.64 | 9.62±1.74 | 0.35 |
V25Gy | 5.18±1.45 | 5.87±0.99 | 1.81 |
Mean dose | 4.87±1.47 | 4.65±1.34 | 0.39 |
WB, proton wobbling; PBS, proton pencil beam scanning.
Tables 11,12 show different Dmax or mean dose of nearby OAR in Group 1 and Group 2. In Group 1, the results are significantly higher in the VMAT photon planning than in the proton treatment planning. Yet in Group 2, the mean dose of the left lung show no difference between the photon and proton plans, and Dmax of esophagus shows different, but photon plans were lower than proton plans. The comparison of the WB and PBS in Group 1 and Group 2 are shown in Tables 13,14 with P value >0.05 in most organs.
Table 11
OAR | Photon (VMAT) | Proton (WB + PBS) | P value |
---|---|---|---|
Esophagus (Dmax) | 1.16±1.33 | 0.0025±0.01 | 0.013* |
Spinal cord (Dmax) | 0.59±0.61 | 0±0 | 0.008* |
LAD | 2.34±2.04 | 0.68±0.675 | 0.009* |
Left lung (mean dose, Gy) | 1.16±0.48 | 0.46±0.32 | 0.005* |
Right lung (mean dose, Gy) | 0.26±0.11 | 0±0 | 0.0001* |
*, P<0.05. OAR, organ at risk; VMAT, photon volumetric modulated arc therapy; WB, proton wobbling; PBS, proton pencil beam scanning; LAD, left anterior descending artery.
Table 12
OAR | Photon (VMAT) | Proton (WB + PBS) | P value |
---|---|---|---|
Esophagus (Dmax) | 18.28±13.77 | 25.16±1.8 | 0.016* |
Spinal cord (Dmax) | 12.23±7.22 | 2.32±4.58 | 0.004* |
LAD | 4.29±3.44 | 0.77±1.47 | 0.030* |
Left lung (mean dose, Gy) | 2.52±1.56 | 2.43±1.43 | 0.426 |
Right lung (mean dose, Gy) | 0.91±0.28 | 0.67±0.30 | 0.062* |
*, P<0.05. OAR, organ at risk; VMAT, photon volumetric modulated arc therapy; WB, proton wobbling; PBS, proton pencil beam scanning; LAD, left anterior descending artery.
Table 13
OAR | WB | PBS | P value |
---|---|---|---|
Esophagus (Dmax) | 0±0 | 0.01±0.01 | 0.17 |
Spinal cord (Dmax) | 0±0 | 0±0 | N/A |
LAD | 0.51±0.71 | 0.84±1.15 | 0.38 |
Left lung (mean dose, Gy) | 0.28±0.27 | 0.65±0.25 | 0.04* |
Right lung (mean dose, Gy) | 0±0 | 0±0 | N/A |
*, P<0.05. OAR, organ at risk; WB, proton wobbling; PBS, proton pencil beam scanning; N/A, not applicable; LAD, left anterior descending artery.
Table 14
OAR | WB | PBS | P value |
---|---|---|---|
Esophagus (Dmax) | 24.78±2.10 | 25.36±1.81 | 0.34 |
Spinal cord (Dmax) | 2.14±3.17 | 2.51±2.79 | 0.41 |
LAD | 1.33±2.10 | 0.21±0.17 | 0.10 |
Left lung (mean dose, Gy) | 2.06±1.55 | 1.74±1.42 | 0.35 |
Right lung (mean dose, Gy) | 0.7±0.33 | 0.65±0.30 | 0.38 |
OAR, organ at risk; WB, proton wobbling; PBS, proton pencil beam scanning; LAD, left anterior descending artery.
Discussion
In a study by Weidlich et al., four different treatment units (CyberKnife, Varian Truebeam, Varian EDGE, Elekta Infinity) were used to compare doses in PTV and OAR for VT patients (25), demonstrating that each was able to deliver a conformal, homogeneous dose to PTV. In addition, the study reported that a conventional linear accelerator was superior in its ability to spare distant critical structures, while CyberKnife showed more effectiveness at sparing nearby critical structures by creating larger dose gradients at the periphery of the target volume. The results are similar to those demonstrated in our study, wherein the HI of the photon and proton are showed no statistical difference in minor at 1.069 and 1.048 respectively, with both being an effective treatment plan. Thus, each treatment plan is able to deliver a conformal, homogeneous dose to the target area.
A study by Knutson et al. in which R50 was used to evaluate dose gradient, reported that the smaller R50 is correlated to a higher dose gradient is (26). A higher dose gradient in the treatment plan is expected to cause lower dose to affect nearby OAR. However, our study revealed no difference in R50 between the photon and proton plans in Group 1 and Group 2, including that the small target causes the dose to decrease rapidly in the photon and proton treatment plans. Generally, the advantage of the proton will be evidenced in lower dose regions, so R30 was also included in our assessment. R30 showed a significant difference only in Group 2. In the photon VMAT planning, as the target region was in center of the heart, beam directions were nearly 200 to 300 degrees in coplanar or non-coplanar, causing a low dose (30% isodose level) region larger than the proton with only 5 beam directions. As shown in Tables 5,6, as compared to the WB technique, PBS has the advantage of reducing the nearby OAR dose by inverse optimization in Group 2, where the location was in the middle of the heart.
Heart toxicity from breast radiotherapy has been a concerning issue for decades. According to a study by Piroth et al. (27), the mean heart dose for breast radiotherapy is recommended as <2.5 Gy, while decreasing the mean heart dose may result in a reduced risk of death caused by radiation-induced heart disease (RIHD) (28). In our study, only the results of the proton treatment plan in Group 1were within the recommend dose, and the results of the proton plan were statistically lower than those of the photon treatment plan. Meanwhile in Group 2, both results exceeded the recommend dose; despite this, the results of the proton plan were still lower than those of photon plan. The study by Piroth et al. further recommends a mean dose of LAD lower than10 Gy. While both Group 1 and Group 2 in our study had a mean dose of LAD lower than 10 Gy, the proton plan remained lower than the photon plan. However, as patients with VT constituted the primary population of the study, it must be noted that the overall survival rate of VT for 1-year and 2-year periods is very low. Thus, in case of VT, the risk of RIHD may not be as concerning as it is in other radiotherapy target.
Figure 2 shows the relationship of volume of CTV and mean heart dose (Gy) in three types of treatment plans. The coefficients of the correlation are 0.61 (P=0.008), 0.474 (P=0.037), 0.471 (P=0.038), revealing a medium correlation in all types of treatment plan and mean heart dose. This indicates that if the mean heart dose is still an index to evaluate harm to OAR, the volume of CTV may be an estimator.
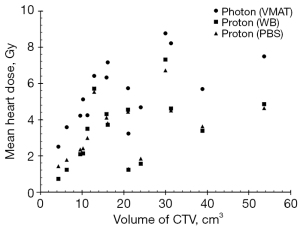
Figure 3 shows the dose volume of heart in the photon plan and proton plans. The results illustrate that the curve of the proton plan is lower than that of the photon plan in all situation except for V20Gy in Group 2. Further analysis reveals that in the volume of the heart receiving a dose greater than 5 Gy and greater than 25 Gy, only V5Gy and V10Gy show a significant difference (Tables 7,9). Although V20Gy is lower in the photon plan than in the proton plan, there is no significant statistical difference. A study by Bradley et al. (29) revealed that a volume of the heart receiving ≥5 or ≥30 Gy was associated with worse overall survival. The proton treatment plan demonstrates the superior overall survival when the heart receives ≥5 Gy and 10 Gy.
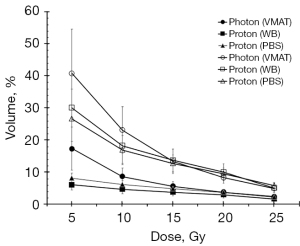
Among the OAR evaluated in this study, the location of the spinal cord, esophagus, and right and left lung are far from PTV in Group 1. Although all have significant differences (P<0.05) between the photon and proton plans, but the values in photon and proton plan are thus not clinically meaningful.
In Group 2, the maximum dose to the esophagus showed no difference between the photon and proton plans, the reason is the location nearby the PTV, and the maximum dose was restricted to under 19 Gy, causing a similar maximum dose in two treatment plans. The proton dose to the left lung and right lung were lower than photon dose, but without significant difference. Of the OAR list, only the spinal cord showed significant difference for the patient with VT occurring at BS, IB, LB. For the location of the three subtracts, the beam angle selection will be more separate than for AA or AI, potentially causing the dose to penetrate the spinal cord given the higher dose than in Group 1, thus, the advantage of the proton is clear.
Conclusions
In this study, the statistical analysis revealed that CI and HI in all treatment plans showed little difference, are sufficient in clinical practice. Although most results indicated that the proton beam will yield less harm to nearby OAR, the advantages of the proton were not significant in some organs in Group 2, in which the VT subtract was located at the center of the heart. Several issues require consideration in future studies, including accurate patient localization, motion margin evaluation of respiration and heartbeat, dose measurement, and verification of small field irradiation. Nevertheless, this treatment planning study indicated that using the proton beam to treat VT is a viable therapeutic option.
Acknowledgments
This study was supported by medical physicist of department of Proton and Radiation Therapy Center, Linkou Chang Gung Memorial Hospital.
Funding: None.
Footnote
Provenance and Peer Review: This article was commissioned by the Guest Editors (Chin-Cheng Chen, Dennis Mah, Chang Chang, Joseph Chang and Nancy Lee) for the series “Pencil Beam Scanning Particle Therapy” published in Therapeutic Radiology and Oncology. The article has undergone external peer review.
Reporting Checklist: The authors have completed the MDAR reporting checklist. Available at https://tro.amegroups.com/article/view/10.21037/tro-21-43/rc
Conflicts of Interest: All authors have completed the ICMJE uniform disclosure form (available at https://tro.amegroups.com/article/view/10.21037/tro-21-43/coif). The series “Pencil Beam Scanning Particle Therapy” was commissioned by the editorial office without any funding or sponsorship. The authors have no other conflicts of interest to declare.
Ethical Statement: The authors are accountable for all aspects of the work in ensuring that questions related to the accuracy or integrity of any part of the work are appropriately investigated and resolved. The study was conducted in accordance with the Declaration of Helsinki (as revised in 2013). The study was approved by Chang Gung Medical Foundation Institutional Review Board (No. 202200668B0) and individual consent for this retrospective analysis was waived.
Open Access Statement: This is an Open Access article distributed in accordance with the Creative Commons Attribution-NonCommercial-NoDerivs 4.0 International License (CC BY-NC-ND 4.0), which permits the non-commercial replication and distribution of the article with the strict proviso that no changes or edits are made and the original work is properly cited (including links to both the formal publication through the relevant DOI and the license). See: https://creativecommons.org/licenses/by-nc-nd/4.0/.
References
- Meissner MD, Akhtar M, Lehmann MH. Nonischemic sudden tachyarrhythmic death in atherosclerotic heart disease. Circulation 1991;84:905-12. [Crossref] [PubMed]
- Sapp JL, Wells GA, Parkash R, et al. Ventricular Tachycardia Ablation versus Escalation of Antiarrhythmic Drugs. N Engl J Med 2016;375:111-21. [Crossref] [PubMed]
- Moss AJ, Hall WJ, Cannom DS, et al. Improved survival with an implanted defibrillator in patients with coronary disease at high risk for ventricular arrhythmia. Multicenter Automatic Defibrillator Implantation Trial Investigators. N Engl J Med 1996;335:1933-40. [Crossref] [PubMed]
- Al-Khatib SM, Stevenson WG, Ackerman MJ, et al. 2017 AHA/ACC/HRS Guideline for Management of Patients With Ventricular Arrhythmias and the Prevention of Sudden Cardiac Death: A Report of the American College of Cardiology/American Heart Association Task Force on Clinical Practice Guidelines and the Heart Rhythm Society. Circulation 2018;138:e272-391. [Crossref] [PubMed]
- Borislav Dinov M, Lukas Fiedler M, Robert Schönbauer M, et al. Outcomes in Catheter Ablation of Ventricular Tachycardia in Dilated Nonischemic Cardiomyopathy Compared With Ischemic Cardiomyopathy. Circulation 2014;129:728-36. [Crossref] [PubMed]
- Tokuda M, Kojodjojo P, Tung S, et al. Acute Failure of Catheter Ablation for Ventricular Tachycardia Due to Structural Heart Disease: Causes and Significance. J Am Heart Assoc 2013;2013;2:e000072.
- Cuculich PS, Schill MR, Kashani R, et al. Noninvasive Cardiac Radiation for Ablation of Ventricular Tachycardia. N Engl J Med 2017;377:2325-36. [Crossref] [PubMed]
- Dusi V, Russo G, Forte GI, et al. Non-invasive ablation of cardiac arrhythmia. Is proton radiation therapy a step forward? Int J Cardiol 2020;313:64-6. [Crossref] [PubMed]
- Goddu SM, Hilliard J, Knutson N, et al. Feasibility of Noninvasive Cardiac Ablation Utilizing Intensity Modulated Proton Therapy to Treat Ventricular Tachycardia. Int J Radiat Oncol Biol Phys 2018;102:S58. [Crossref]
- Lehmann HI, Graeff C, Simoniello P, et al. Feasibility Study on Cardiac Arrhythmia Ablation Using High-Energy Heavy Ion Beams. Sci Rep 2016;6:38895. [Crossref] [PubMed]
- Benedict SH, Yenice KM, Followill D, et al. Stereotactic body radiation therapy: the report of AAPM Task Group 101. Med Phys 2010;37:4078-101. [Crossref] [PubMed]
- Kim EJ, Davogustto G, Stevenson WG, et al. Non-invasive Cardiac Radiation for Ablation of Ventricular Tachycardia: a New Therapeutic Paradigm in Electrophysiology. Arrhythm Electrophysiol Rev 2018;7:8-10. [Crossref] [PubMed]
- Bert C, Engenhart-Cabillic R, Durante M. Particle therapy for noncancer diseases. Med Phys 2012;39:1716-27. [Crossref] [PubMed]
- Lehmann HI, Deisher AJ, Takami M, et al. External Arrhythmia Ablation Using Photon Beams: Ablation of the Atrioventricular Junction in an Intact Animal Model. Circ Arrhythm Electrophysiol 2017;10:e004304. [Crossref] [PubMed]
- Constantinescu A, Lehmann HI, Packer DL, et al. Treatment Planning Studies in Patient Data With Scanned Carbon Ion Beams for Catheter-Free Ablation of Atrial Fibrillation. J Cardiovasc Electrophysiol 2016;27:335-44. [Crossref] [PubMed]
- de Riva M, Watanabe M, Zeppenfeld K. Twelve-lead ECG of ventricular tachycardia in structural heart disease. Circ Arrhythm Electrophysiol 2015;8:951-62. [Crossref] [PubMed]
- Ohe Tohru, Shimomura Katsuro, Alhara Naohiko, et al. Idiopathic sustained left ventricular tachycardia: clinical and electrophysiologic characteristics. Circulation 1988;77:560-8. [Crossref] [PubMed]
- Haqqani HM, Tschabrunn CM, Tzou WS, et al. Isolated septal substrate for ventricular tachycardia in nonischemic dilated cardiomyopathy: incidence, characterization, and implications. Heart Rhythm 2011;8:1169-76. [Crossref] [PubMed]
- Haqqani HM, Callans DJ. Ventricular Tachycardia in Coronary Artery Disease. Cardiac Electrophysiology Clinics 2014;6:525-34. [Crossref]
- Tzou WS, Zado ES, Lin D, et al. Sinus rhythm ECG criteria associated with basal-lateral ventricular tachycardia substrate in patients with nonischemic cardiomyopathy. J Cardiovasc Electrophysiol 2011;22:1351-8. [Crossref] [PubMed]
- Xiao Y, Kry SF, Popple R, et al. Flattening filter-free accelerators: a report from the AAPM Therapy Emerging Technology Assessment Work Group. J Appl Clin Med Phys 2015;16:5219. [Crossref] [PubMed]
- Feuvret L, Noel G, Mazeron JJ, et al. Conformity index: a review. Int J Radiat Oncol Biol Phys 2006;64:333-42. [Crossref] [PubMed]
- Kataria T, Sharma K, Subramani V, et al. Homogeneity Index: An objective tool for assessment of conformal radiation treatments. J Med Phys 2012;37:207-13. [Crossref] [PubMed]
- Chan M, Wong M, Leung R, et al. Optimizing the prescription isodose level in stereotactic volumetric-modulated arc radiotherapy of lung lesions as a potential for dose de-escalation. Radiat Oncol 2018;13:24. [Crossref] [PubMed]
- Weidlich GA, Hacker F, Bellezza D, et al. Ventricular Tachycardia: A Treatment Comparison Study of the CyberKnife with Conventional Linear Accelerators. Cureus 2018;10:e3445. [Crossref] [PubMed]
- Knutson NC, Samson PP, Hugo GD, et al. Radiation Therapy Workflow and Dosimetric Analysis from a Phase 1/2 Trial of Noninvasive Cardiac Radioablation for Ventricular Tachycardia. Int J Radiat Oncol Biol Phys 2019;104:1114-23. [Crossref] [PubMed]
- Piroth MD, Baumann R, Budach W, et al. Heart toxicity from breast cancer radiotherapy: Current findings, assessment, and prevention. Strahlenther Onkol 2019;195:1-12. [Crossref] [PubMed]
- Filopei J, Frishman W. Radiation-induced heart disease. Cardiol Rev 2012;20:184-8. [Crossref] [PubMed]
- Bradley JD, Paulus R, Komaki R, et al. Standard-dose versus high-dose conformal radiotherapy with concurrent and consolidation carboplatin plus paclitaxel with or without cetuximab for patients with stage IIIA or IIIB non-small-cell lung cancer (RTOG 0617): a randomised, two-by-two factorial phase 3 study. Lancet Oncol 2015;16:187-99. [Crossref] [PubMed]
Cite this article as: Wang HT, Wu LS, Chou YC, Yeh YS, Yen KC, Chang JTC, Huang HC. Feasibility assessment of proton treatment for ventricular tachycardia—treatment planning study. Ther Radiol Oncol 2022;6:17.