Dosimetric comparison of breath-hold and free-breathing techniques in left-sided breast cancer patients treated with proton pencil beam scanning
Introduction
Breast cancer is the most common malignancy diagnosed in women in both the United States and worldwide, with an estimated incidence of 281,550 cases in 2021 in the United States (1,2). In appropriately selected patients, adjuvant radiation therapy (RT) reduces the risk of locoregional and distant failure after both breast-conserving surgery and mastectomy, leading to a reduction in breast cancer mortality (3-11).
The survival benefit provided in this setting is limited by dose to surrounding organs at risk (OAR), especially the heart, as multiple studies have shown a proportional risk of cardiovascular disease with increasing doses to the heart (12,13). Photon irradiation, particularly for left-sided breast cancer, may increase the risk of cardiac disease in some patients due to elevated cardiac exposure (14-16).
Breath-hold techniques are commonly employed when treating breast cancer patients with photon irradiation in order to reduce dose to the heart and lungs by creating increased separation between the chest wall and the normal tissue which lies posterior to it, as is visualized in Figure 1 (17,18). There are several drawbacks and limitations to the use of breath-hold techniques, including certain patients’ difficulty tolerating the procedure, others deriving minimal anatomical benefit, and a longer duration of treatment, which affects patient convenience and clinic workflow.
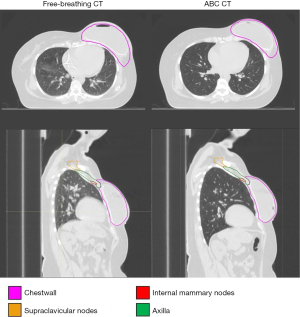
Due to its unique depth-dose distribution with rapid fall off beyond the Bragg peak, proton therapy (PT) provides a means to improve the therapeutic ratio by reducing exposure to surrounding OAR within the thorax, such as the heart, lungs, contralateral breast, bones, and dissected axilla, without sacrificing target coverage (19-23). Correspondingly, PT has the potential to decrease the risk of cardiac disease, pneumonitis, lymphedema, fracture, drop in blood counts, and secondary malignancy. Proton irradiation has been shown to decrease cardiac and lung dose in comparison with photon RT delivered via 3D-conformal and IMRT modalities with both free-breathing (FB) and breath-hold techniques (24-27). A systematic review published by Taylor et al. in 2015 showed an average heart dose of 8 Gy with photon RT when including the internal mammary lymph nodes in comparison with only 2.6 Gy radiobiological equivalent (GyE) with protons (28). As the developing technology and understanding of RT delivery has improved, heart dose has further diminished with both photon and proton irradiation, with more recent studies reporting mean heart doses of 0.5–1.0 GyE with PT (27,29-33).
The benefit of breath-hold techniques with PT is unclear. This is in part due to the rapid dose fall off of protons, which are less affected by anatomical differences posterior to the target when treating with en face beams with PT as opposed to tangents with photon RT. There is no established standardized approach for the use of breath-hold techniques with PT, highlighted by the protocol for the ongoing Radiotherapy Comparative Effectiveness (RADCOMP) Consortium trial (NCT02603341) comparing proton and photon modalities for breast cancer, which allows both FB and breath-hold techniques based on physician preference (34).
We compared heart and lung doses resulting from breath-hold and FB intensity-modulated proton therapy (IMPT) treatment plans in patients with left-sided breast cancer. We hypothesized that lung dose would be significantly higher in patients treated with breath-hold due to a reduction in lung density compared to FB IMPT plans, while cardiac doses would be similar with the two techniques. We present this article in accordance with the MDAR and STROBE reporting checklists (available at https://tro.amegroups.com/article/view/10.21037/tro-22-5/rc).
Methods
Patient selection
Eight patients with node positive left-sided breast cancer treated at The Johns Hopkins Proton Center who underwent IMPT between September 2020 and August 2021 on a protocol for retrospective data analysis approved by the institutional review board (IRB #00284297) were included in accordance with the Declaration of Helsinki (as revised in 2013). These included 7 post-mastectomy patients and 1 breast conservation patient. Four of these patients were treated after breast expander (n=3) or silicone implant reconstruction (n=1) and 4 were treated without reconstruction. The Informed Consent and Health Insurance Portability and Accountability Act (HIPAA) authorization were waived, given that it was a retrospective chart review and the fact that no patient health information was reported.
Imaging and treatment planning
All patients underwent active breathing control (ABC) coaching prior to simulation to ensure consistent breath-holds. Patients were simulated on a wingboard with arms abducted above the head. Wire was placed on all scars and 2 cm below the inframammary border/scar, and ball bearings (BBs) were placed at anticipated field edges. Patients were scanned from the chin to 10 cm below the inframammary wire/scar with 2 mm slice thickness. A FB planning computerized tomography (CT) simulation was acquired in addition to three CTs with ABC.
Diagnostic magnetic resonance imaging (MRI) and Fluciclovine F18 positron emission tomography (PET) imaging were fused to CT simulation scans when available. All clinical target volume (CTV) and OAR contours were delineated by the attending radiation oncologist in the RayStation 10A treatment planning system (RaySearch Laboratories, Stockholm, Sweden). CTVs included the chest wall for post-mastectomy patients and whole breast for breast-conservation patients, in addition to axillary, infraclavicular, supraclavicular, and internal mammary nodal stations, with the posterior neck excluded in all patients. CTV contours were delineated according to the RADCOMP trial protocol (NCT02603341) (34). Contoured OARs included the heart, left anterior descending (LAD) artery, ipsilateral and contralateral lungs, spinal canal, esophagus, thyroid, ipsilateral brachial plexus, and ipsilateral humeral head.
The dose was 50 GyE over 25 fractions, prescribed to the CTV using a radiological equivalent value of 1.1 (35). RayStation 10A was used to create plans using 2–3 (0°–10°, 30°–40°, 60°) treatment fields with a range shifter. Proton plans consisted of IMPT using pencil beam scanning with discrete spot scanning. The nominal spot size in σ, as defined at the isocenter in air (~110 MeV), was 4.15 mm, with spot spacing ratio of 0.6 used for planning.
Treatment plans were created on ABC scans for clinical treatment and re-planned on FB scans for analysis. Inverse optimization was used to generate appropriate dose distribution with pre-specified weighting of target coverage and OAR sparing using modulation of beam spot location, energy and weight. All plans were calculated with robustness using single field optimization with a setup uncertainty of 5 mm and range uncertainty of 3.5% using fast graphics processing units (GPU) Monte Carlo optimization. ABC plans were evaluated to ensure coverage on all three acquired ABC simulation CTs. Dosimetric goals for CTV coverage and OAR constraints are provided in Table 1, as defined by our institutional standards. Mean lung density was defined as the mean Hounsfield unit (HU) value of the CT intensities within the left-lung contour.
Table 1
Targets/OAR | Parameter | Goal | Constraint |
---|---|---|---|
CTV | V95% | >95% | >90% |
V53.5GyE | <50 cc | <200 cc | |
Ipsilateral lung | V5GyE | <35% | <40% |
V20GyE | <16% | <20% | |
Contralateral lung | V5GyE | <10% | < 15% |
Total lungs | V20GyE | <10% | <15% |
Heart | V5GyE | <5% | <7% |
V20GyE | <1% | <2% | |
Max dose† | <25 GyE | <25 GyE | |
Mean dose | <1 GyE | <2 GyE | |
Spinal canal | Max dose† | <45 GyE | <45 GyE |
Body | Max dose† | <55 GyE | <57.5 GyE |
Thyroid | V30GyE | <66% | <66% |
Mean dose | <20 GyE | <20 GyE | |
Esophagus | Max dose† | <40 GyE | <45 GyE |
Mean dose | <20 GyE | <30 GyE | |
Ipsilateral humeral head | Max dose† | <50 GyE | <50 GyE |
Ipsilateral brachial plexus | Max dose† | <57.5 GyE | <60 GyE |
Skin | Max dose† | <47.5 GyE | <47.5 GyE |
†, maximum dose to 0.03 cc. OAR, organs at risk; CTV, clinical target volume; V95%, volume receiving 95% of prescription (i.e., 47.5 GyE); GyE, radiobiological Gy equivalent; V53.5GyE, volume receiving 53.5 GyE; V5GyE, volume receiving 5 GyE; V20GyE, volume receiving 20 GyE; V30GyE, volume receiving 30 GyE; cc, cubic centimeters.
Statistical analysis
All statistical analyses were performed in SPSS 26.0 (IBM, Armonk, NY, USA). Paired t-tests were used to determine the differences between ABC and FB lung, heart, and LAD doses, lung density, and time on table for beam. Dosimetric differences between patients treated with and without implant reconstruction were also analyzed using paired t-tests for both ABC and FB. Results were considered significant when the probability of making a type I error was less than 5% (P<0.05).
Results
ABC and FB CT images of a representative patient are provided in Figure 1, which shows a lower CT intensity (less dense) within the lung in the ABC scan compared to the FB scan. While the appearance of the lungs on these images can depend on the windowing used, an objective statistical comparison of the two breathing techniques showed that ipsilateral lung density was significantly lower in ABC CT scans than in FB CT scans (−805 vs. −661 HU, P<0.01), as shown in Table 2.
Table 2
Parameters | ABC (n=8) | FB (n=8) | P value |
---|---|---|---|
Lung density (HU) | −805 (31) | −661 (65) | <0.01* |
Lung mass density (g/cm3) | 0.22 (0.03) | 0.37 (0.07) | <0.01* |
CTV V95% (%) | 97.0 (3.4) | 96.8 (3.2) | 0.75 |
Ipsilateral lung V5GyE (%) | 34.9 (7.9) | 29.1 (7.2) | <0.01* |
Ipsilateral lung V20GyE (%) | 13.9 (2.3) | 10.4 (2.3) | <0.01* |
Contralateral lung V5GyE (%) | 3.2 (2.9) | 2.4 (2.6) | 0.04* |
Heart V5GyE (%) | 2.9 (2.5) | 5.2 (4.2) | 0.06 |
Heart V20GyE (%) | 0.1 (0.1) | 0.8 (0.9) | 0.06 |
Heart V30GyE (%) | 0.0 (0.0) | 0.2 (0.2) | 0.06 |
Heart V40GyE (%) | 0.0 (0.0) | 0.0 (0.0) | 0.14 |
Heart V50GyE (%) | 0.0 (0.0) | 0.0 (0.0) | 0.35 |
Heart max dose (GyE)† | 24.8 (8.3) | 35.8 (11.9) | 0.03* |
Heart mean dose (GyE) | 0.6 (0.4) | 0.9 (0.7) | 0.06 |
LAD artery V15GyE (%) | 9.1 (10.7) | 19.5 (14.6) | 0.15 |
LAD artery V30GyE (%) | 0.3 (0.7) | 1.7 (2.1) | 0.15 |
LAD artery V40GyE (%) | 0.0 (0.0) | 0.2 (0.4) | 0.21 |
Time on table for beam (min) | 13.8 (4.9) | 7.6 (2.0) | 0.01* |
Data are present as mean (SD). *, considered statistically significant based on P<0.05; †, maximum dose to 0.03 cc. ABC, active breathing control; FB, free breathing; HU, Hounsfield unit; CTV, clinical target volume; V95%, volume receiving 95% of prescription (i.e., 47.5 GyE); GyE, radiobiological Gy equivalent; V5GyE, volume receiving 5 GyE; V20GyE, volume receiving 20 GyE; V30GyE, volume receiving 30 GyE; V40GyE, volume receiving 40 GyE; V50GyE, volume receiving 50 GyE; LAD, left anterior descending; V15GyE, volume receiving 15 GyE; SD, standard deviation; cc, cubic centimeters.
Figure 2 shows two representative patients with and without breast reconstruction. In both cases, it is visually apparent that coverage is consistent between the plans optimized on the ABC and FB scan. It is also apparent that the lung dose is higher in the ABC scans, particularly the 5 GyE isodose line, which covers more volume of lung in the ABC CT compared to the FB CT.
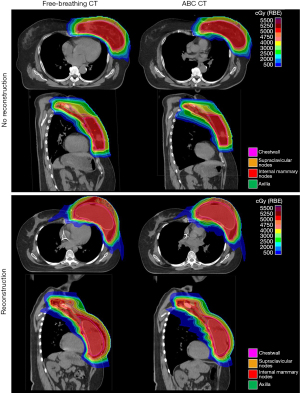
A comparison of FB and ABC is provided for each endpoint in Table 2. CTV coverage was not significantly different between ABC and FB plans (97.0% vs. 96.8%, P=0.75). Volumes of ipsilateral lung receiving 5 GyE (34.9% vs. 29.1%, P<0.01) and 20 GyE (13.9% vs. 10.4%, P<0.01) were significantly higher with ABC than with FB. Volume of contralateral lung receiving 5 GyE was significantly higher with ABC than with FB (3.2% vs. 2.4%, P=0.04). Maximum heart dose was lower with ABC than FB (24.8 vs. 35.8 GyE, P=0.03), but there were no statistically significant differences in any other heart or LAD volumetric endpoint between ABC and FB, including mean heart dose, volume of heart receiving 5, 20, 30, 40, and 50 GyE, and volume of LAD receiving 15, 30, and 40 GyE. Volume of heart receiving 5 GyE for FB plans exceeded the goal of <5%, but did not exceed the constraint of <7%. All other CTV and OAR goals were achieved by both ABC and FB plans. Time on table for beam was significantly longer with ABC than with FB treatment (13.8 vs. 7.6 min, P=0.01).
A comparison between patients with and without reconstruction for both ABC and for FB plans is provided in Table 3. Mean heart dose and the volume of heart receiving 5 GyE were significantly higher in reconstructed patients than those who had not undergone reconstruction in both ABC and FB plans. Volume of heart receiving 20 GyE was higher in reconstructed patients than those who had not undergone reconstruction in ABC plans but not in FB plans. Volume of lung receiving 5 GyE was slightly higher in reconstructed patients for both FB and ABC plans than the goal of 35% but did not exceed the constraint of 40%.
Table 3
Parameters | Reconstruction (n=4) | No Reconstruction (n=4) | P value |
---|---|---|---|
ABC | |||
CTV V95% (%) | 95.5 (4.4) | 98.6 (0.9) | 0.21 |
Ipsilateral lung V5GyE (%) | 36.7 (5.7) | 33.1 (10.2) | 0.57 |
Ipsilateral lung V20GyE (%) | 14.4 (1.2) | 13.3 (3.2) | 0.54 |
Contralateral lung V5GyE (%) | 3.7 (3.5) | 2.7 (2.6) | 0.66 |
Heart V5GyE (%) | 4.9 (1.8) | 0.9 (0.8) | <0.01* |
Heart V20GyE (%) | 0.2 (0.1) | 0.0 (0.0) | 0.03* |
Heart V30GyE (%) | 0.0 (0.0) | 0.0 (0.0) | 0.39 |
Heart V40GyE (%) | 0.0 (0.0) | 0.0 (0.0) | – |
Heart V50GyE (%) | 0.0 (0.0) | 0.0 (0.0) | – |
Heart max dose (GyE)† | 28.1 (4.5) | 21.5 (10.5) | 0.18 |
Heart mean dose (GyE) | 0.8 (0.3) | 0.3 (0.1) | <0.01* |
LAD artery V15GyE (%) | 16.0 (11.3) | 2.1 (2.6) | 0.08 |
LAD artery V30GyE (%) | 0.6 (1.0) | 0.0 (0.0) | 0.35 |
LAD artery V40GyE (%) | 0.0 (0.0) | 0.0 (0.0) | – |
FB | |||
CTV V95% (%) | 95.3 (3.9) | 98.4 (1.5) | 0.19 |
Ipsilateral lung V5GyE (%) | 30.3 (6.9) | 27.8 (8.3) | 0.66 |
Ipsilateral lung V20GyE (%) | 11.1 (1.3) | 9.7 (3.1) | 0.44 |
Contralateral lung V5GyE (%) | 3.2 (2.8) | 1.5 (2.5) | 0.41 |
Heart V5GyE (%) | 8.6 (3.2) | 1.8 (0.7) | <0.01* |
Heart V20GyE (%) | 1.4 (0.9) | 0.2 (0.2) | 0.05 |
Heart V30GyE (%) | 0.3 (0.3) | 0.1 (0.1) | 0.11 |
Heart V40GyE (%) | 0.0 (0.1) | 0.0 (0.0) | 0.48 |
Heart V50GyE (%) | 0.0 (0.0) | 0.0 (0.0) | 0.39 |
Heart max dose (GyE)† | 36.8 (12.3) | 34.7 (13.3) | 0.87 |
Heart mean dose (GyE) | 1.5 (0.6) | 0.4 (0.1) | 0.01* |
LAD artery V15GyE (%) | 26.7 (16.6) | 12.4 (9.3) | 0.23 |
LAD artery V30GyE (%) | 0.8 (1.1) | 2.5 (2.7) | 0.42 |
LAD artery V40GyE (%) | 0.0 (0.0) | 0.4 (0.5) | 0.23 |
Data are present as mean (SD). *, considered statistically significant based on P<0.05; †, maximum dose to 0.03 cc. ABC, active breathing control; CTV, clinical target volume; V95%, volume receiving 95% of prescription (i.e., 47.5 GyE); GyE, radiobiological Gy equivalent; V5GyE, volume receiving 5 GyE; V20GyE, volume receiving 20 GyE; V30GyE, volume receiving 30 GyE; V40GyE, volume receiving 40 GyE; V50GyE, volume receiving 50 GyE; LAD, left anterior descending; V15GyE, volume receiving 15 GyE; FB, free breathing; SD, standard deviation; cc, cubic centimeters.
Discussion
In this study, we evaluated dosimetric values for CTV coverage and OAR sparing in patients treated with IMPT for left-sided breast cancer. We made the following observations: (I) with similar target coverage, maximum heart dose was higher with FB than with ABC, but no other cardiac dose parameters were significantly different between FB and ABC CT plans; (II) lung dose was significantly higher in ABC plans; and (III) the beam on time was significantly longer with ABC treatments than with FB treatments.
The results from this study show mostly similar dosimetric values for CTV coverage and OAR dose for ABC and FB plans, with slightly higher volumes of lung receiving 5 and 20 GyE with ABC plans and similar cardiac doses with both techniques outside of maximum heart dose. Our results are concordant with other studies which have investigated differences between breath-hold and FB techniques in proton irradiation for patients with left-sided breast cancer (36-39). The dosimetric plans provided in this analysis were all created using Monte Carlo optimization, which is not the nationwide or global standard. This has been shown to provide a higher accuracy than other dose calculation methods.
We found similarly low mean heart doses with FB and breath-hold techniques. A dosimetric comparative study between FB and deep inspiration breath hold (DIBH) techniques was conducted by Speleers et al., which found a significant decrease in mean cardiac dose with DIBH vs. FB when treating with proton irradiation (40). However, this study treated all patients in the prone position using anterior oblique beams, limiting extrapolation to our cohort of patients treated supine with en face beams.
Studies which included proton plans generated in a similar fashion to the techniques employed in our cohort, using pencil beam scanning proton beams delivered en face, found comparable results as those shown in our study, with no significant difference in dose to heart and lungs with the addition of breath hold. Yu et al. compared breath hold to FB techniques when treating left-sided breast cancer using scanning beam protons with various beam arrangements, from tangents to en face (39). They found that breath hold did not decrease heart dose, with tangent beams providing inferior target coverage when using FB techniques. Patel et al. compared dosimetric outcomes between four planning techniques when treating with left-sided post-mastectomy radiation, including tangent photons with DIBH, passively scattered protons during FB, and pencil beam scanning protons with and without DIBH (37). While all three proton plans decreased dose to the heart and lungs in comparison with photon plans, there was no significant differences in dose to the heart and lungs between any of the proton techniques, regardless of the use of DIBH.
While mean heart dose and all heart and LAD volumetric endpoints were similar between the two breathing techniques, maximum heart dose was higher with FB than ABC. Certain dosimetric endpoints have been shown to correlate with cardiac toxicity, including mean heart dose, volume of heart receiving 5, 30, and 40 GyE, and volume of LAD receiving 15 GyE (12,13,41-44). These parameters are likely more strongly associated with clinical outcomes such as overall survival and major adverse cardiac events than maximum heart point dose. Thus, while it is possible that an increase in maximum heart dose may be clinically significant, the lack of difference between the two breathing techniques in any of the aforementioned mean and volumetric endpoints suggests that FB and ABC likely provide equivalent risk of cardiac toxicity.
The increase in lung dose with ABC may be partially explained by the understanding that as the lungs expand with maximal inspiration techniques, they become less dense, providing less proton stopping power. This can cause the proton beam to range further into the lung. This may explain why the uncertainty of proton stopping power is higher in lung than bone or soft tissue (45). This was corroborated by our results, which showed significantly higher lung density with FB scans than with ABC scans.
While the benefit provided by ABC may be minimal when treating with en face beams, the differences between the two modalities may be larger when treating with tangential beams, which are more sensitive to the respiratory motion inherent to the FB approach (39). Thus, caution should be taken before extrapolating our results to scenarios in which tangential beams are used for treatment.
The time on table for beam, during which breath hold must be maintained for ABC cases, was significantly longer with ABC than with FB plans (13.8 vs. 7.6 min). The task of holding one’s breath cumulatively for over 13 min per treatment is worth highlighting, as this can be quite taxing for patients. This does not capture the additional time required for ABC coaching, ABC CT simulation, and the time on table when the beam is off, which is significant when treating with IMPT due to the large number of breath-holds required for each treatment. Thus, the time discrepancy between the two techniques is much larger than captured in our results.
There is limited data comparing dosimetric outcomes for patients with and without reconstruction receiving proton irradiation. We found that several heart dosimetric endpoints were higher in patients with reconstruction than those without, regardless of breathing technique. Outcomes using PT for breast cancer in patients with and without reconstruction were reported in studies by Macdonald et al. and Depauw et al., however comparisons of differences between the two populations and comparisons of breathing techniques were not included in these studies (29,31).
We initially conjectured that cardiac doses might be higher in patients with expanders as a result of overcoming the density of the metal expander valve, however the cardiac dose was also higher in the patient who was treated with a silicone implant. Given the small number of patients in each cohort, it is difficult to make meaningful conclusions from these results.
Motion with respiration and dosimetric impact
One question that arises relates to the interplay between the motion of the breast with respiration during FB and temporal delivery of scanning beam PT via spot scanning. This has been investigated for lung tumors (46-51). It has been studied in the post-mastectomy setting in a study by Depauw et al., where the investigators evaluated the averaged dosimetric effect with respiratory motion over multiple fractions (31). It has also been studied in the setting of accelerated partial-breast irradiation, which showed that IMPT plans using tangential beams were very sensitive to respiratory motion, while en face beams had less deterioration of target coverage with DIBH (52).
Differences in cardiac dose between FB and DIBH have also been studied when treating left-sided breast cancer in the setting of breast-conserving surgery and post-mastectomy, showing that respiratory motion during FB did not have a significant impact on dosimetry when treating with en face beams and that BH did not significantly reduce any cardiac dosimetric endpoints (39). A study by Klaassen et al. showed that the effect of breathing motion on robustness is minimal when treating left-sided breast cancer patients with proton irradiation (53).
Limitations
None of the patients included in this study were treated with hypofractionated courses of radiation. While hypofractionation has increasingly become the standard of care in the treatment of breast cancer, its use with PT in the post-mastectomy setting has not been established in prospective fashion to date. We await results of ongoing trials such as that being conducted at Mayo Clinic (NCT02783690).
Our institution is in the process of implementing and testing the benefits of dual energy CT. None of the patients treated in this study underwent dual energy CT simulation. Dual energy CT would better predict stopping power by reducing the uncertainty of tissue composition variation through its ability to simultaneously detect effective atomic number and electron density (54). This is an evolving portion of the field which has potential to further benefit our understanding of dose deposition in the future.
Conclusions
Breath-hold plans provided slightly higher lung dose and mostly similar cardiac dose in comparison with plans using FB techniques in the treatment of left sided breast cancer with PT. Given the large increase in the time required for breath-hold treatment without obvious dosimetric or clinical benefit, FB techniques may be preferred in order to maximize clinic workflow and minimize patient inconvenience, particularly in patients without cardiac risk factors.
Acknowledgments
Funding: None.
Footnote
Provenance and Peer Review: This article was commissioned by the Guest Editors (Chin-Cheng Chen, Dennis Mah, Chang Chang, Joseph Chang and Nancy Lee) for the series “Pencil Beam Scanning Particle Therapy” published in Therapeutic Radiology and Oncology. The article has undergone external peer review.
Reporting Checklist: The authors have completed the MDAR and STROBE reporting checklists. Available at https://tro.amegroups.com/article/view/10.21037/tro-22-5/rc
Conflicts of Interest: All authors have completed the ICMJE uniform disclosure form (available at https://tro.amegroups.com/article/view/10.21037/tro-22-5/coif). The series “Pencil Beam Scanning Particle Therapy” was commissioned by the editorial office without any funding or sponsorship. The authors have no other conflicts of interest to declare.
Ethical Statement: The authors are accountable for all aspects of the work in ensuring that questions related to the accuracy or integrity of any part of the work are appropriately investigated and resolved. The study was conducted in accordance with the Declaration of Helsinki (as revised in 2013). The study was approved by the Institutional Review Board (IRB #00284297) and individual consent was waived, given that it was a retrospective chart review and the fact that no patient health information was reported.
Open Access Statement: This is an Open Access article distributed in accordance with the Creative Commons Attribution-NonCommercial-NoDerivs 4.0 International License (CC BY-NC-ND 4.0), which permits the non-commercial replication and distribution of the article with the strict proviso that no changes or edits are made and the original work is properly cited (including links to both the formal publication through the relevant DOI and the license). See: https://creativecommons.org/licenses/by-nc-nd/4.0/.
References
- American Cancer Society. Cancer facts and figures, 2021. In: Book Cancer facts and figures, 2021. Atlanta: American Cancer Society, 2021.
- Ferlay J, Soerjomataram I, Dikshit R, et al. Cancer incidence and mortality worldwide: sources, methods and major patterns in GLOBOCAN 2012. Int J Cancer 2015;136:E359-86. [Crossref] [PubMed]
- Early Breast Cancer Trialists' Collaborative Group (EBCTCG). Effect of radiotherapy after breast-conserving surgery on 10-year recurrence and 15-year breast cancer death: meta-analysis of individual patient data for 10,801 women in 17 randomised trials. Lancet 2011;378:1707-16. [Crossref] [PubMed]
- Fisher B, Anderson S, Bryant J, et al. Twenty-year follow-up of a randomized trial comparing total mastectomy, lumpectomy, and lumpectomy plus irradiation for the treatment of invasive breast cancer. N Engl J Med 2002;347:1233-41. [Crossref] [PubMed]
- EBCTCG (Early Breast Cancer Trialists' Collaborative Group). Effect of radiotherapy after mastectomy and axillary surgery on 10-year recurrence and 20-year breast cancer mortality: meta-analysis of individual patient data for 8135 women in 22 randomised trials. Lancet 2014;383:2127-35. [Crossref] [PubMed]
- Clarke M, Collins R, Darby S, et al. Effects of radiotherapy and of differences in the extent of surgery for early breast cancer on local recurrence and 15-year survival: an overview of the randomised trials. Lancet 2005;366:2087-106. [Crossref] [PubMed]
- Whelan TJ, Olivotto IA, Levine MN. Regional Nodal Irradiation in Early-Stage Breast Cancer. N Engl J Med 2015;373:1878-9. [Crossref] [PubMed]
- Poortmans PM, Collette S, Kirkove C, et al. Internal Mammary and Medial Supraclavicular Irradiation in Breast Cancer. N Engl J Med 2015;373:317-27. [Crossref] [PubMed]
- Overgaard M, Hansen PS, Overgaard J, et al. Postoperative radiotherapy in high-risk premenopausal women with breast cancer who receive adjuvant chemotherapy. Danish Breast Cancer Cooperative Group 82b Trial. N Engl J Med 1997;337:949-55. [Crossref] [PubMed]
- Overgaard M, Jensen MB, Overgaard J, et al. Postoperative radiotherapy in high-risk postmenopausal breast-cancer patients given adjuvant tamoxifen: Danish Breast Cancer Cooperative Group DBCG 82c randomised trial. Lancet 1999;353:1641-8. [Crossref] [PubMed]
- Thorsen LB, Offersen BV, Danø H, et al. DBCG-IMN: A Population-Based Cohort Study on the Effect of Internal Mammary Node Irradiation in Early Node-Positive Breast Cancer. J Clin Oncol 2016;34:314-20. [Crossref] [PubMed]
- Darby SC, Ewertz M, McGale P, et al. Risk of ischemic heart disease in women after radiotherapy for breast cancer. N Engl J Med 2013;368:987-98. [Crossref] [PubMed]
- Dess RT, Sun Y, Matuszak MM, et al. Cardiac Events After Radiation Therapy: Combined Analysis of Prospective Multicenter Trials for Locally Advanced Non-Small-Cell Lung Cancer. J Clin Oncol 2017;35:1395-402. [Crossref] [PubMed]
- Correa CR, Litt HI, Hwang WT, et al. Coronary artery findings after left-sided compared with right-sided radiation treatment for early-stage breast cancer. J Clin Oncol 2007;25:3031-7. [Crossref] [PubMed]
- Darby SC, McGale P, Taylor CW, et al. Long-term mortality from heart disease and lung cancer after radiotherapy for early breast cancer: prospective cohort study of about 300,000 women in US SEER cancer registries. Lancet Oncol 2005;6:557-65. [Crossref] [PubMed]
- Marks LB, Yu X, Prosnitz RG, et al. The incidence and functional consequences of RT-associated cardiac perfusion defects. Int J Radiat Oncol Biol Phys 2005;63:214-23. [Crossref] [PubMed]
- Smyth LM, Knight KA, Aarons YK, et al. The cardiac dose-sparing benefits of deep inspiration breath-hold in left breast irradiation: a systematic review. J Med Radiat Sci 2015;62:66-73. [Crossref] [PubMed]
- Latty D, Stuart KE, Wang W, et al. Review of deep inspiration breath-hold techniques for the treatment of breast cancer. J Med Radiat Sci 2015;62:74-81. [Crossref] [PubMed]
- MacDonald SM, Jimenez R, Paetzold P, et al. Proton radiotherapy for chest wall and regional lymphatic radiation; dose comparisons and treatment delivery. Radiat Oncol 2013;8:71. [Crossref] [PubMed]
- Xu N, Ho MW, Li Z, et al. Can proton therapy improve the therapeutic ratio in breast cancer patients at risk for nodal disease? Am J Clin Oncol 2014;37:568-74. [Crossref] [PubMed]
- Bradley JA, Dagan R, Ho MW, et al. Initial Report of a Prospective Dosimetric and Clinical Feasibility Trial Demonstrates the Potential of Protons to Increase the Therapeutic Ratio in Breast Cancer Compared With Photons. Int J Radiat Oncol Biol Phys 2016;95:411-21. [Crossref] [PubMed]
- Lin LL, Vennarini S, Dimofte A, et al. Proton beam versus photon beam dose to the heart and left anterior descending artery for left-sided breast cancer. Acta Oncol 2015;54:1032-9. [Crossref] [PubMed]
- Wilson RR. Radiological use of fast protons. Radiology 1946;47:487-91. [Crossref] [PubMed]
- Loap P, Tkatchenko N, Goudjil F, et al. Cardiac substructure exposure in breast radiotherapy: a comparison between intensity modulated proton therapy and volumetric modulated arc therapy. Acta Oncol 2021;60:1038-44. [Crossref] [PubMed]
- Mondal D, Jhawar SR, Millevoi R, et al. Proton versus Photon Breath-Hold Radiation for Left-Sided Breast Cancer after Breast-Conserving Surgery: A Dosimetric Comparison. Int J Part Ther 2021;7:24-33. [Crossref] [PubMed]
- Lee HL, Lim LH, Master Z, et al. The role of breath hold intensity modulated proton therapy for a case of left-sided breast cancer with IMN involvement. How protons compare with other conformal techniques? Tech Innov Patient Support Radiat Oncol 2020;15:1-5. [Crossref] [PubMed]
- Mast ME, Vredeveld EJ, Credoe HM, et al. Whole breast proton irradiation for maximal reduction of heart dose in breast cancer patients. Breast Cancer Res Treat 2014;148:33-9. [Crossref] [PubMed]
- Taylor CW, Wang Z, Macaulay E, et al. Exposure of the Heart in Breast Cancer Radiation Therapy: A Systematic Review of Heart Doses Published During 2003 to 2013. Int J Radiat Oncol Biol Phys 2015;93:845-53. [Crossref] [PubMed]
- MacDonald SM, Patel SA, Hickey S, et al. Proton therapy for breast cancer after mastectomy: early outcomes of a prospective clinical trial. Int J Radiat Oncol Biol Phys 2013;86:484-90. [Crossref] [PubMed]
- Jimenez RB, Goma C, Nyamwanda J, et al. Intensity modulated proton therapy for postmastectomy radiation of bilateral implant reconstructed breasts: a treatment planning study. Radiother Oncol 2013;107:213-7. [Crossref] [PubMed]
- Depauw N, Batin E, Daartz J, et al. A novel approach to postmastectomy radiation therapy using scanned proton beams. Int J Radiat Oncol Biol Phys 2015;91:427-34. [Crossref] [PubMed]
- Ares C, Khan S, Macartain AM, et al. Postoperative proton radiotherapy for localized and locoregional breast cancer: potential for clinically relevant improvements? Int J Radiat Oncol Biol Phys 2010;76:685-97. [Crossref] [PubMed]
- Cuaron JJ, Chon B, Tsai H, et al. Early toxicity in patients treated with postoperative proton therapy for locally advanced breast cancer. Int J Radiat Oncol Biol Phys 2015;92:284-91. [Crossref] [PubMed]
- Bekelman JE, Lu H, Pugh S, et al. Pragmatic randomised clinical trial of proton versus photon therapy for patients with non-metastatic breast cancer: the Radiotherapy Comparative Effectiveness (RadComp) Consortium trial protocol. BMJ Open 2019;9:e025556. [Crossref] [PubMed]
- Wambersie A. RBE, reference RBE and clinical RBE: applications of these concepts in hadron therapy. Strahlenther Onkol 1999;175:39-43. [Crossref] [PubMed]
- Ranger A, Dunlop A, Hutchinson K, et al. A Dosimetric Comparison of Breast Radiotherapy Techniques to Treat Locoregional Lymph Nodes Including the Internal Mammary Chain. Clin Oncol (R Coll Radiol) 2018;30:346-53. [Crossref] [PubMed]
- Patel SA, Lu HM, Nyamwanda JA, et al. Postmastectomy radiation therapy technique and cardiopulmonary sparing: A dosimetric comparative analysis between photons and protons with free breathing versus deep inspiration breath hold. Pract Radiat Oncol 2017;7:e377-84. [Crossref] [PubMed]
- Flejmer AM, Chehrazi B, Josefsson D, et al. Impact of physiological breathing motion for breast cancer radiotherapy with proton beam scanning - An in silico study. Phys Med 2017;39:88-94. [Crossref] [PubMed]
- Yu J, Park SS, Herman MG, et al. Free Breathing versus Breath-Hold Scanning Beam Proton Therapy and Cardiac Sparing in Breast Cancer. Int J Part Ther 2017;3:407-13. [Crossref] [PubMed]
- Speleers B, Schoepen M, Belosi F, et al. Effects of deep inspiration breath hold on prone photon or proton irradiation of breast and regional lymph nodes. Sci Rep 2021;11:6085. [Crossref] [PubMed]
- Atkins KM, Chaunzwa TL, Lamba N, et al. Association of Left Anterior Descending Coronary Artery Radiation Dose With Major Adverse Cardiac Events and Mortality in Patients With Non-Small Cell Lung Cancer. JAMA Oncol 2021;7:206-19. [Crossref] [PubMed]
- Bradley JD, Paulus R, Komaki R, et al. Standard-dose versus high-dose conformal radiotherapy with concurrent and consolidation carboplatin plus paclitaxel with or without cetuximab for patients with stage IIIA or IIIB non-small-cell lung cancer (RTOG 0617): a randomised, two-by-two factorial phase 3 study. Lancet Oncol 2015;16:187-99.
- Chun SG, Hu C, Choy H, et al. Impact of Intensity-Modulated Radiation Therapy Technique for Locally Advanced Non-Small-Cell Lung Cancer: A Secondary Analysis of the NRG Oncology RTOG 0617 Randomized Clinical Trial. J Clin Oncol 2017;35:56-62. [Crossref] [PubMed]
- Taylor C, Correa C, Duane FK, et al. Estimating the Risks of Breast Cancer Radiotherapy: Evidence From Modern Radiation Doses to the Lungs and Heart and From Previous Randomized Trials. J Clin Oncol 2017;35:1641-9. [Crossref] [PubMed]
- Yang M, Zhu XR, Park PC, et al. Comprehensive analysis of proton range uncertainties related to patient stopping-power-ratio estimation using the stoichiometric calibration. Phys Med Biol 2012;57:4095-115. [Crossref] [PubMed]
- Li Y, Kardar L, Li X, et al. On the interplay effects with proton scanning beams in stage III lung cancer. Med Phys 2014;41:021721. [Crossref] [PubMed]
- Kardar L, Li Y, Li X, et al. Evaluation and mitigation of the interplay effects of intensity modulated proton therapy for lung cancer in a clinical setting. Pract Radiat Oncol 2014;4:e259-68. [Crossref] [PubMed]
- Grassberger C, Dowdell S, Lomax A, et al. Motion interplay as a function of patient parameters and spot size in spot scanning proton therapy for lung cancer. Int J Radiat Oncol Biol Phys 2013;86:380-6. [Crossref] [PubMed]
- Li H, Liu W, Park P, et al. Evaluation of the systematic error in using 3D dose calculation in scanning beam proton therapy for lung cancer. J Appl Clin Med Phys 2014;15:4810. [Crossref] [PubMed]
- Seco J, Robertson D, Trofimov A, et al. Breathing interplay effects during proton beam scanning: simulation and statistical analysis. Phys Med Biol 2009;54:N283-94.
- Li H, Li Y, Zhang X, et al. Dynamically accumulated dose and 4D accumulated dose for moving tumors. Med Phys 2012;39:7359-67. [Crossref] [PubMed]
- Wang X, Zhang X, Li X, et al. Accelerated partial-breast irradiation using intensity-modulated proton radiotherapy: do uncertainties outweigh potential benefits? Br J Radiol 2013;86:20130176. [Crossref] [PubMed]
- Klaassen L, Petoukhova AL, Habraken SJM, et al. Effect of breathing motion on robustness of proton therapy plans for left-sided breast cancer patients with indication for locoregional irradiation. Acta Oncol 2021;60:222-8. [Crossref] [PubMed]
- Yang M, Virshup G, Clayton J, et al. Theoretical variance analysis of single- and dual-energy computed tomography methods for calculating proton stopping power ratios of biological tissues. Phys Med Biol 2010;55:1343-62. [Crossref] [PubMed]
Cite this article as: Gogineni E, Fellows Z, Hrinivich WT, Li H, Croog V, Wright JL, Sheikh K. Dosimetric comparison of breath-hold and free-breathing techniques in left-sided breast cancer patients treated with proton pencil beam scanning. Ther Radiol Oncol 2023;7:6.